Translate this page into:
Comparison of antimicrobial effect of selenium nanoparticles and silver nanoparticles coated orthodontic mini-implants – An in vitro study
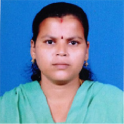
*Corresponding author: Prema Anbarasu, Department of Orthodontics, Chettinad Dental College and Research Institute, Chennai, Tamil Nadu, India. prema.arasu@gmail.com
-
Received: ,
Accepted: ,
How to cite this article: Subramanian SK, Anbarasu P, Navin N, Iyer SR. Comparison of antimicrobial effect of selenium nanoparticles and silver nanoparticles coated orthodontic mini-implants – An in vitro study. APOS Trends Orthod 2022;12:20-6.
Abstract
Objectives:
Mini-implants have earned a significant role in orthodontic treatment, by augmenting anchorage requirements. Peri-implantitis contributes to miniscrew failures where progressive peri-implant bone loss occurs in conjunction with soft-tissue inflammation due to the growth of microorganisms such as Streptococcus and Lactobacillus. Nanoparticles have increased surface area and have increased interactions with biological targets like bacteria. This study aims to investigate the antimicrobial activity of silver nanoparticles (AgNP) and selenium nanoparticles (SeNPs) on orthodontic mini-implants.
Material and Methods:
Mini-implant (Ti-6Al-4V) was coated with AgNP and SeNP with biopolymer (Ti-BPAgNP and Ti-BPSeNP) by dip-coating technique. The crystal structure and crystallite size of AgNPs and SeNPs were characterized by the X-ray diffraction (XRD) method. The size distribution and morphology of SeNP and AgNP were determined by a scanning electron microscope (SEM). The antibacterial activity of Ti-BP-AgNP and Ti-BPSeNP was detected from the zone of inhibition by disk diffusion assay.
Results:
The SEM image of AgNP was roughly spherical, uniformly distributed and SeNPs were spherical, well distributed on the biopolymer surface. The area of the zone of inhibition of Ti-BP-SeNP-coated mini-implants shows a negligible difference in antibacterial activity compared to Ti-BPAgNP-coated mini-implants.
Conclusion:
Ti-BP-AgNP and Ti-BP-SeNP showed that a strong antibacterial activity was against Lactobacillus and Staphylococcus aureus. Antibacterial activity against Streptococcus mutans was slightly less than observed in other bacteria. SeNP shows only a marginal difference in antibacterial activity when compared to AgNP.
Keywords
Nanoparticles
Silver
Selenium
Mini-implants
Orthodontics
INTRODUCTION
Mini-implants have earned a significant position in orthodontic treatment, in augmenting anchorage requirements by overpowering the limitations of conventional extraoral and intraoral anchorage appliances. Mini-implants are the temporary anchorage device fixed temporarily on the bone to amplify orthodontic anchorage either by supporting the teeth of the reactive unit or by obviating the need for the reactive unit altogether and are subsequently removed after use. They can be placed transosteal, endosteal, or subperiosteal and are fixed to bone either biochemically (osseointegration) or mechanically (cortical stabilization).[1]
The mini-implants can be placed in areas where conventional orthodontic appliances or natural anchorage are impractical, such as the palate, the zygomatic process, the edentulous spaces in the alveolus of either arch, the ramus, and the retromolar region. They provide firm anchorage to move multiple teeth. They can be used to intrude or extrude teeth, close edentulous spaces, reposition malposed teeth, treat vertical malocclusion, correct undesirable malocclusion, provides orthopedic anchorage, and reinforce anchorage.[2] In comparison to traditional anchorage systems, TADs provide greater patient comfort, simpler orthodontic mechanics, shorter treatment time, no patient dependence, and only minor anchorage loss.[3,4]
The stability of the implant determines the success rate which depends on the site of insertion, the bone quality, soft tissues surrounding the insertion site, and maintenance of the implant. Peri-implantitis contributes to 30% of miniscrew failures where progressive peri-implant bone loss occurs in conjunction with soft-tissue inflammation.[5] Patients with poor oral hygiene act as a nidus for the growth of microorganisms such as Streptococcus species and Lactobacillus over the site of placement of mini-implants.[6]
In orthodontics, silver nanoparticles (AgNPs) have been incorporated in orthodontic wires, adhesives, composites, brackets, bands, and modules, and in mini-implants, as they have an antibacterial effect. Similar Ti-AgNPs also possess antibacterial properties. In dentistry, studies on selenium nanoparticles (SeNP) are limited. Hence, SeNP can be attempted in dental implants due to their antibacterial potential.[7] Selenium is essential for protecting cells and tissues against oxidative damage in the human body.[8] For optimal functioning of the immune system, elemental Se is required, particularly in controlling the behavior of macrophages.[9] SeNPs play a role in influencing the macrophage signal transduction pathways induced by the bacterial endotoxin and lipopolysaccharide.[10] NPs have increased surface area and have increased interactions with biological targets like bacteria. Hence, this study aims to investigate the antimicrobial activity of AgNP and SeNPs on orthodontic mini-implants.
MATERIAL AND METHODS
Mini-implant specifications
Shape of the head: button overall length – 9.8 mm, thread length – 6.0 mm, and thread diameter – 1.4 mm. Composition: Wrought Titanium-6 Aluminium-4 Vanadium ELI (Ti-6Al-4V) (Dentos Inc., Korea). Before use, mini-implants were sealed in individual sterilized pouches (Dentos Inc., Korea).
Synthesis of AgNP and SeNPs
Synthesis of AgNP and SeNPs is done using the conventional method.[11] A 200 ml of 0.001 M of silver nitrate dissolved in 500 ml of distilled water was mixed with 200 ml of 0.01 M glucose dissolved in 500 ml of distilled water in a beaker for 2 min at 60°C using a magnetic stirrer. Then, 100 ml of 0.01 M sodium citrate was added and stirred. The reaction resulted in a solution color change from milky white to brown and finally a gray solution after 16 h. The mixture thus obtained was washed with distilled water to attain neutral pH and it is centrifuged. It was then washed with acetone and centrifuged at 6000 rpm for 5 min. The gray precipitate obtained was dried in a Petri dish and a dry powder of AgNPs was obtained.
A 60 ml of 25 mM of sodium selenite, 60 ml of 100 mM glutathione, and 3 g of bovine serum albumin were dissolved in 180ml of double-distilled water. After mixing the reactant solution, the pH of the solution was adjusted to alkaline media by adding 1 ml NaOH to the reactant solution visualized by a color change from clear white to a red color solution. Then, SeNPs were obtained by centrifuging (cooling centrifuge) the solution at 13,000 rpm with 30 min duration at −4°C temperature which are air-dried and collected as dry powder.
Coating of biopolymer and NPs over mini-implants
Biopolymer: Acrylonitrile Butadiene Styrene (ABS)
A 1 ml of chloroform was taken in two small closed tubes and ABS polymer is dispersed in it and soaked well to get it dissolved over the chloroform. Later, AgNP and SeNP were dissolved in these separate tubes by sonication process. Once the gel phase is attained the head portion of the mini-implant was held and coating of the gel (nanoparticle biopolymer) was done by dip-coating technique. After completion of the biopolymer-AgNP deposition and biopolymer-SeNP deposition on the mini-implant surface, the color of the mini-implant had turned blackish discoloration and brownish-gold, respectively.[12]
Crystal structure and crystallite size of AgNPs and SeNPs were characterized by the X-ray diffraction method (XRD) (Miniflex 600). To determine the phase distribution, crystallinity, and purity of synthesized NPs, XRD analysis is used. The generator current and voltage were set at 25 mA and 35 KV. The AgNP and SeNP samples were scanned in the continuous scan mode in the 2θ range at 20–80°C and 0.04°/s scan rate.
The size distribution and morphology of SeNP and AgNP and the shape of the AgNPs that were synthesized by the chemical reduction method were determined by scanning electron microscope (SEM) at DR. VB’s Ceramic Research Center, Chennai.
Bacterial culture preparation and disk diffusion assay
Staphylococcus aureus ATCC 29213, Streptococcus mutans ATCC 10449, and Lactobacillus ATCC 4356 were used for the antibacterial effect assay. The bacterial suspensions were prepared from saline suspensions of isolated colonies selected from the nutrient broth. Then, the nutrient broth was cultured for about 24 h. The suspension was adjusted to match the tube of 0.5 McFarland turbidity standard using a spectrophotometer at 600 nm, equated to 1.5 × 108 colony-forming unit/ml. Mueller-Hinton agar plates were prepared. A sterile swab was completely soaked in the prepared bacterial suspension which was used to inoculate the surface of the Mueller-Hinton agar plates. Finally, the AgNP- and SeNP-coated mini-implants were placed on the inoculated agar plate and it is incubated at 37°C for 24 h. After incubation, the breadth, length, and area of the inhibition zones were measured and tabulated.
RESULTS
XRD analysis of AgNP and SeNPs
The characteristic face-centered cubic silver lines correspond to all diffraction peaks. These diffraction lines observed at 2θ angles 32.8°, 38.2°, 55.10°, and 65.7° were indexed, respectively, as (111), (200), (220), and (311). The crystal size was analyzed using the Scherer formula, D=βλ/FWHM(2θ) cosθ. FWHM is the full width of the peak, 2θ is the scattering angle in radians, λ is the wavelength, b is a constant, which normally takes a value between 0.89 and 0.94 depending on the function used to fit the peak, and D is the dimension of the crystallites. D is interpreted for different reflections and different crystallite shapes. The standard XRD pattern has shown that the sample contains AgNPs in a mixed-phase (cubic and hexagonal) structure [Figure 1]. The generator current and voltage were set at 25 mA and 35 KV. The diffraction peaks at 2θ angles 36.7, 42.8, 63.2, and 76.4 were indexed as (100), (101), (110), and (102) reflections of the pure hexagonal phase of Se crystals with lattice parameters a=4.366A0 and c=4.9536A0 (JC PDS Card No 06-362) [Figure 2].

- X-ray diffraction analysis of silver nanoparticle.

- X-ray diffraction analysis of selenium nanoparticle.
SEM analysis of AgNP and SeNP with biopolymer coated mini-implants (Ti-BP-AgNP) and (Ti-BP-SeNP)
SEM image of the silver-coated biopolymer surfaces confirmed the development of AgNPs over the mini-implants showing a clear image of highly dense AgNPs [Figure 3]. SEM results at the magnification of 50 μm shows AgNPs roughly spherical and were uniformly distributed in the biopolymer-coated implant. SEM images of the selenium-coated biopolymer surfaces showed that the SeNPs were roughly spherical and were well distributed on the biopolymer surface at the magnification of 50 μm confirmed the development of SeNPs and were seen over the mini-implants [Figure 4].

- Scanning electron microscope image of silver nanoparticle.

- Scanning electron microscope image of selenium nanoparticle.
Antibacterial activity – disk diffusion assay
The antibacterial activity of the AgNP-coated and SeNP-coated mini-implants was confirmed by the presence of a zone of inhibition of the growth of S. mutans, S. aureus, and Lactobacillus around the substrate [Figures 5 and 6]. No bacterial growth was observed around AgNP-coated and SeNP-coated mini-implants. However, bacterial growth was seen around the uncoated group (control). Strong antibacterial activity was observed against Lactobacillus and S. aureus. Antibacterial activity against S. mutans was detected, but the inhibition zone was less than that observed in other bacteria.

- Disk diffusion tests showing zone of inhibition for (a) Streptococcus mutans with Ti-BP-AgNP; (b) Lactobacillus with Ti-BP-AgNP; and (c) Staphylococcus aureus with Ti-BP-AgNP.

- Disk diffusion tests showing zone of inhibition for (a) Streptococcus mutans with Ti-BP-SeNP; (b) Lactobacillus with Ti-BP-SeNP; and (c) Staphylococcus aureus with Ti-BP-SeNP.
The area of the zone of inhibition of Ti-BP-SeNP coated mini-implants shows less antibacterial activity compared to Ti-BP-AgNP coated mini-implants which denote the antibacterial activity of SeNP was less compared to the AgNP [Table 1]. However, there were no zones of inhibition seen in the control [Figure 7].
Bacteria | Zone of inhibition | ||||||||
---|---|---|---|---|---|---|---|---|---|
Ti-BP-AgNP | Ti-BP-SeNP | Control | |||||||
Length | Breadth | Area | Length | Breadth | Area | Length | Breadth | Area | |
Staphylococcus aureus | 22.15±0.9 | 11.75±0.44 | 204.41±0.31 | 15.67±0.42 | 10.89±0.53 | 134.03±0.17 | - | - | - |
Lactobacillus | 16.45±0.54 | 7.63±0.37 | 98.58±0.15 | 13.76±0.67 | 6.98±0.39 | 75.43±0.21 | - | - | - |
Streptococcus mutans | 13.68±0.61 | 5.87±0.27 | 63.07±0.13 | 12.25±0.54 | 4.57±0.23 | 43.97±0.09 | - | - | - |
AgNP: Silver nanoparticle, SeNP: Selenium nanoparticle

- (a) Macroscopic image of coated and uncoated implants and (b) disk diffusion tests showing zone of inhibition of uncoated implants (control).
DISCUSSION
Mini-implants have been widely used in orthodontics as absolute anchorage and are typically loaded early to minimize the duration of treatment and removed after treatment.[13] Failures were associated with inadequate oral hygiene and persistent inflammation. The mere existence of fixed orthodontic devices makes oral hygiene difficult and can alter the nature of bacterial plaque in orthodontic patients, causing environmental changes in the oral cavity. Poor attention to oral hygiene has resulted in tissue inflammation around mini-implants and hastened their loss.[14]
In our study, the AgNPs and SeNPs were incorporated with biopolymer to improve the antimicrobial characteristics. The SEM images showed AgNPs and SeNPs dispersed over the coated mini-implant surface with a diameter of approximately 50–80 nm. To verify the array of chemical elements, XRD analyzes were performed and confirmed with diffraction peaks at 2θ ranges.
NPs have shown wide-spectrum antibacterial properties against bacteria that are both Gram-negative and Gram-positive. In medicine and dentistry, the use of some NPs as antimicrobial agents has gained a lot of interest. NPs are considered to be insoluble particles of a dimension smaller than 100 nm. NPs are deposited on titanium mini-implants in the field of implantology and have proved to be useful for both cell proliferations and to prevent infection from microorganism.[15]
In orthodontic patients, there is a reported increase in Streptococcus sp. and Lactobacillus, so these common pathogens were considered appropriate for evaluating the antibacterial test.[16] The results of our study show that both AgNP and SeNP have antibacterial activity with only a marginal difference in antibacterial activity of SeNP when compared to the increased antibacterial activity of AgNP. Strong antibacterial activity was observed against Lactobacillus and S. aureus when compared to S. mutans. The results are similar to the study by Sreenivasagan et al., where mini-implant coated with chitosan-AgNPs showed a strong antibacterial effect against Lactobacillus and S. aureus whereas antibacterial activity obtained against S. mutans was minimal but no antifungal activity against Candida albicans.[17]
The antibacterial function of AgNPs revolves around a lot of speculation. The potential of these NPs to release ionic silver in an aqueous solution is correlated with several hypotheses describing this phenomenon.[18] Some are linked to their ability to respond with the bacterial cell membrane and others are linked to their ability to produce reactive oxygen species.[19] Most significantly, because of their large surface area, AgNPs are known to elicit antimicrobial properties, which, in turn, allow for a larger area for microbial contact. Silver ions also have a strong affinity toward electron donor groups present in various oxygen-, nitrogen-, or sulfur-containing bacterial cells.[20]
Selenium salts when used in large quantities are toxic, but trace amounts of selenium are essential for normal cellular function in many organisms. In humans and animals, selenium is naturally present as part of selenoproteins, which play an important role in thyroid hormone metabolism, antioxidant defense systems, and redox control of cell reactions. SeNPs have great potential as a wide-spectrum antimicrobial agent against bacteria, fungi, viruses, and parasites.[21] The biomedical application of the antibacterial properties of SeNP has focused on preventing implanted medical devices from bacterial colonization.
The results of Sreenivasagan et al. in 2020 complement the findings of our study which shows that titanium mini-implants coated with AgNP have excellent antimicrobial properties.[17]
In 2017, Adith Venugopal et al. displayed excellent antibacterial properties in titanium microimplants by modifying the surface of orthodontic microimplants with biopolymer-AgNP coating, whereas no antibacterial effect was observed in the uncoated group.[22] The study documented the importance of coating biopolymer over the orthodontic miniscrews. In Ti-AgNp miniscrew group, only 1.05% atomic silver were only sparsely dispersed, whereas, in Ti-BP-AgNP miniscrews group, AgNPs were densely dispersed over the miniscrews with 21.2% atomic silver.
In addition, the polymers have a synergistic impact on NPs when used in combination, although the antibacterial performance of polymers alone is much lower than that of NPs.[23] Therefore, the NPs can be stabilized and dispelled by biopolymers, preventing their oxidation and thus increasing their bioavailability to enhance their antibacterial properties. With their substantial antibacterial properties, NPs play a crucial role in the immediate antibacterial impact of nanoparticle-deposited biopolymer coatings.
In the study by Adith Venugopal et al., hydroxyapatite/ chitosan biopolymer was used which can enhance bone formation resulting in increased osseointegration around the mini-implant.[22] For osseointegrated mini-implants, the torque used to remove mini-implant should be made easy to prevent breakage of mini-implant or damage to the adjacent anatomical structure.[24] Some researchers have also reported loosening of the coating through progressive crystallization of the HA that causes stress accumulation within the coating, especially under load in bone.[25]
To prevent osseointegration of hydroxyapatite/chitosan biopolymer, ABS polymer has been used in our study. ABS polymer has high dimensional stability, high rigidity, good abrasion resistance, stain resistance, and stability over a long time. Junior et al., in their study, concluded that ABS polymer is inert and biocompatible and it is well tolerated to tissue.[26]
Zhang et al. highlighted the key cellular effects mediated by AgNPs, such as cell uptake and intracellular dissemination, cytotoxicity, genotoxicity, and immune responses, as well as some of the main in vitro and in vivo factors influencing these effects, such as dosage, time, scale, shape, surface chemistry, and cell type.[27] After exposure to AgNPs, in vivo biodistribution studies have documented local and distant organ accumulation and toxicity of Ag.[28]
SeNPs with lower cytotoxic effects than those of AgNPs has gained considerable interest. Conclusive reports on the role of scale, monodispersity, surface charge, morphology, etc., on their antibacterial efficacy, however, are scarce. SeNPs have reported antimicrobial properties against common hospital pathogens, particularly Pseudomonas aeruginosa and S. aureus.[29] In orthodontics, selenium-containing orthodontic material was used to prevent white spot lesions with antimicrobial properties against S. mutans and Lactobacillus acidophilus.[30] Liu et al. discussed that SeNPtitanium dioxide nanotubes exhibited antibacterial and anti-inflammatory properties to enhance the efficiency of titanium-based implants for various orthopedics and dental applications.[7]
Most investigators found that selenium has potent antibacterial and anti-inflammatory properties. Vennila et al. had shown that SeNP has potent anticancer activity against human cervical cancer cells, antibacterial, and anti-inflammatory activity in comparison to the AgNP.[31] Similarly, the incorporation of SeNPs and AgNPs into furcellaran-gelatin (FUR/GEL) films was stated by Jamróz et al. that SeNP-containing FUR/GEL films displayed higher antimicrobial activity against Escherichia coli, S. aureus, and MRSA than AgNP-containing films.[32] It can, therefore, be concluded that SeNPs can be a promising candidate for the AgNP substitution in the forthcoming antibacterial applications.
A thorough literature search in the medical database reveals that this is the first study reporting a fabrication of a biopolymer coated with AgNP and SeNP over titanium mini-implants to enumerate the antibacterial effects of AgNP and SeNPs and a comparison of AgNPs with SeNPs to understand the difference in antibacterial effects of both. Future research on the sustainability of the release of ions from these nanoparticle-coated biopolymers, transitions in the physical properties of the deposited material, such as long-term oral stability, clinically applicable retention of the biopolymer coating, and most significantly, oral tissue toxicity should be carried out to justify its use as a suitable implantable biomaterial.
CONCLUSION
Ti-BP-AgNP and Ti-BP-SeNP showed a strong antibacterial activity/against Lactobacillus and S. aureus. Antibacterial activity against S. mutans was also detected, but the zone of inhibition was slightly less when compared to other bacteria.
SeNP shows only a marginal decrease in the antibacterial effect when compared to the AgNP.
SeNP with lower cytotoxic effects and effective antibacterial activity can be used as the best antibacterial substitute in the forthcoming antibacterial applications in implantology.
Declaration of patient consent
Patient consent is not required as there are no patients in this study.
Financial support and sponsorship
Nil.
Conflicts of interest
There are no conflicts of interest.
References
- Mini-implants in the anchorage armamentarium: New paradigms in the orthodontics. Int J Biomater. 2012;2012:394121.
- [CrossRef] [PubMed] [Google Scholar]
- Dental implants for orthodontic anchorage. Am J Orthod Dentofac Orthop. 2005;127:713-22.
- [CrossRef] [PubMed] [Google Scholar]
- Comparison of the effects of mini-implant and traditional anchorage on patients with maxillary dentoalveolar protrusion. Angle Orthod. 2017;87:320-7.
- [CrossRef] [PubMed] [Google Scholar]
- Effectiveness of orthodontic miniscrew implants in anchorage reinforcement during en-masse retraction: A systematic review and meta-analysis. Am J Orthod Dentofac Orthop. 2017;151:440-55.
- [CrossRef] [PubMed] [Google Scholar]
- Factors associated with the stability of mini-implants for orthodontic anchorage: A study of 414 samples in Taiwan. J Oral Maxillofac Surg. 2009;67:1595-9.
- [CrossRef] [PubMed] [Google Scholar]
- Plaque retention by self-ligating vs elastomeric orthodontic brackets: Quantitative comparison of oral bacteria and detection with adenosine triphosphate-driven bioluminescence. Am J Orthod Dentofac Orthop. 2009;135:426-e1.
- [CrossRef] [Google Scholar]
- Selenium nanoparticles incorporated into Titania nanotubes inhibit bacterial growth and macrophage proliferation. Nanoscale. 2016;8:15783-94.
- [CrossRef] [PubMed] [Google Scholar]
- Investigation of functional selenium nanoparticles as potent antimicrobial agents against superbugs. Acta Biomater. 2016;30:397-407.
- [CrossRef] [PubMed] [Google Scholar]
- NF-κB signaling pathway, not IFN-β/STAT1, is responsible for the selenium suppression of LPS-induced nitric oxide production. Int Immunopharmacol. 2007;7:1192-8.
- [CrossRef] [PubMed] [Google Scholar]
- Selenium attenuates pro-inflammatory gene expression in macrophages. Mol Nutr Food Res. 2008;52:1316-23.
- [CrossRef] [PubMed] [Google Scholar]
- Preparation of sliver and selenium nanoparticles and its characterization by dynamic light scattering and scanning electron microscopy. J Microsc Ultrastruct. 2018;6:182.
- [Google Scholar]
- Surface and structural properties of medical acrylonitrile butadiene styrene modified with silver nanoparticles. Polymers. 2020;12:197.
- [CrossRef] [PubMed] [Google Scholar]
- Mini-implants in orthodontics: A systematic review of the literature. Am J Orthod Dentofac Orthop. 2009;135:564-e1.
- [CrossRef] [PubMed] [Google Scholar]
- Microbial colonization in orthodontic mini-implants. Braz Dent J. 2012;23:422-7.
- [CrossRef] [PubMed] [Google Scholar]
- The antimicrobial activity of nanoparticles: Present situation and prospects for the future. Int J Nanomed. 2017;12:1227.
- [CrossRef] [PubMed] [Google Scholar]
- Periodontopathogens around the surface of mini-implants removed from orthodontic patients. Angle Orthod. 2012;82:591-5.
- [CrossRef] [PubMed] [Google Scholar]
- Assessment of antimicrobial activity and cytotoxic effect of green mediated silver nanoparticles and its coating onto mini-implants. Ann Phytomed. 2020;9:207-12.
- [CrossRef] [Google Scholar]
- Silver nanoparticle enhanced silver ion stress response in Escherichia coli K12. Nanotoxicology. 2012;6:857-66.
- [CrossRef] [PubMed] [Google Scholar]
- Role of reactive oxygen species in the antibacterial mechanism of silver nanoparticles on Escherichia coli O157: H7. Biometals. 2012;25:45-53.
- [CrossRef] [PubMed] [Google Scholar]
- Silver nanoparticles: Synthesis, antimicrobial coatings, and applications for medical devices. Recent Patents Mater Sci. 2015;8:166-75.
- [CrossRef] [Google Scholar]
- Biogenic selenium nanoparticles: Current status and future prospects. Appl Microbiol Biotechnol. 2016;100:2555-66.
- [CrossRef] [PubMed] [Google Scholar]
- Incorporation of silver nanoparticles on the surface of orthodontic microimplants to achieve antimicrobial properties. Korean J Orthod. 2017;47:3-10.
- [CrossRef] [PubMed] [Google Scholar]
- Surface-grafted viologen for precipitation of silver nanoparticles and their combined bactericidal activities. Langmuir. 2004;20:6847-52.
- [CrossRef] [PubMed] [Google Scholar]
- Removal torque of osseointegrated mini-implants: An in vivo evaluation. Eur J Orthod. 2007;29:443-8.
- [CrossRef] [PubMed] [Google Scholar]
- Microstructural changes in bone of HA-coated implants. J Biomed Mater Res. 1998;39:23-31.
- [CrossRef] [Google Scholar]
- Tissue reaction and integration of polyamide and acrylonitrilebutadiene-styrene spheres in rat subcutaneous tissue. Braz J Vet Med. 2016;38(Suppl 1):93-8.
- [Google Scholar]
- Changes in oral health-related quality of life during fixed orthodontic appliance therapy. Am J Orthod Dentofac Orthop. 2008;133:25-9.
- [CrossRef] [PubMed] [Google Scholar]
- Health impact of silver nanoparticles: A review of the biodistribution and toxicity following various routes of exposure. Int J Mol Sci. 2020;21:2375.
- [CrossRef] [PubMed] [Google Scholar]
- Antibacterial effect of the laser-generated Se nanocoatings on Staphylococcus aureus and Pseudomonas aeruginosa biofilms. Laser Phys Lett. 2017;15:15604.
- [CrossRef] [Google Scholar]
- Antimicrobial effect of orthodontic materials on cariogenic bacteria Streptococcus mutans and Lactobacillus acidophilus. Med Sci Monit Basic Res. 2020;26:e920510-1.
- [CrossRef] [Google Scholar]
- Comparison of biological activities of selenium and silver nanoparticles attached with bioactive phytoconstituents: Green synthesized using Spermacoce hispida extract. Adv Nat Sci. 2018;9:15005.
- [CrossRef] [Google Scholar]
- Development and characterisation of furcellaran-gelatin films containing SeNPs and AgNPs that have antimicrobial activity. Food Hydrocoll. 2018;83:9-16.
- [CrossRef] [Google Scholar]