Translate this page into:
Comparison of stress relaxation properties between 3-dimensional printed and thermoformed orthodontic aligners: A pilot study of in vitro simulation of two consecutive 8-hours force application
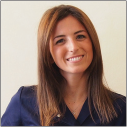
*Corresponding author: Francesca Cremonini, Postgraduate School of Orthodontics, University of Ferrara, Ferrara, Italy. dr.ssafrancescacremonini@gmail.com
-
Received: ,
Accepted: ,
How to cite this article: Cremonini F, Brucculeri L, Pepe F, Palone M, Lombardo L. Comparison of stress relaxation properties between 3-dimensional printed and thermoformed orthodontic aligners: A pilot study of in vitro simulation of two consecutive 8-hours force application. APOS Trends Orthod. 2024;14:225-34. doi: 10.25259/APOS_201_2023
Abstract
Objectives:
The study is aimed to examine the stress relaxation of 3d-printed aligners, Tera Hartz TC-85 (Graphy Inc. Seoul, South Korea) and Polyamide (Noxi, Sweden and Martina, Due Carrare, Padova, Italy), in comparison to thermoformed aligners, Zendura FLX (Bay Materials LLC, Fremont, California, USA) and Duran (SCHEU, Iserlohn, Germany).
Material and Methods:
A stress-relaxation test was conducted using a motorized vertical testing bench, TVO-S (AstraLab, Mariano Comense, Italy), applying a constant and controlled pre-set load. Each sample was subjected to a deflection of 0.5 mm while being immersed in water for eight consecutive h at a constant temperature of 37°C. During this period, data were collected at intervals of 1 s. The same test was repeated twice (test 1 and test 2) on the same specimen, in order to simulate the intermittence of deflection force.
Results:
For both Test 1 and Test 2, a total of 28,800 measurements were recorded for each aligner, with one measurement taken per second over a duration of 8 h/test. A statistically significant difference (P < 0.001) was found for all materials and at all time periods were considered. If the percentage of stress relaxation is considered, a significant difference among the four aligners was found as well.
Conclusion:
All materials displayed substantial stress decay during the 8-h period of constant load, although significant differences were observed among the various materials under investigation. The Noxi aligner demonstrated the highest force values in both tests, confirmed by low percentages of stress relaxation ranging from 23% to 32%.
Keywords
3D-printed
Clear aligners
Stress relaxation
Materials
INTRODUCTION
Clear orthodontic aligners made their debut in the late 1990s and have witnessed a recent surge in popularity, especially among adult patients seeking orthodontic treatment.[1]
Aligner materials consist of resin polymers that are not inert and can undergo changes when exposed to warmth, humidity, mastication forces, and prolonged contact with salivary enzymes in the oral environment.[2] Manufacturers primarily use polyethylene terephthalate glycol (PET-G) and thermoplastic polyurethanes (TPUs) in a single-layer of thickness which typically ranges from 0.50 mm to 1.5 mm.[3,4] Nowadays, various multi-layer orthodontic clear aligners, generally 3-layer combination, are available with significantly better biomechanical properties compared to single-layer ones.[5]
The clear aligner materials offer several advantages, including improved esthetics and comfort, while being equally effective as fixed appliances for treating mild-to-moderate malocclusions.[6] However, it is important to acknowledge the drawbacks of clear aligners. If the environmental impact is considered, this kind of treatment has high costs, both in the production of the aligners themselves and the models of the arches on which they need to be thermoformed.[7]
Furthermore, if the efficiency of the treatment in moderate-severe malocclusions is considered, a high number of aligners is necessary. thus leading to prolonged treatment times; Therefore, to achieve satisfactory results in some selected cases, may be comparable to those of fixed appliances.[8-10]
While various factors can influence the clinical effectiveness of clear aligners, the properties of the materials used in their fabrication remain crucial in determining their mechanical and clinical characteristics.[11,12]
A physical three-dimensional (3D)-printed stereolithography model is required to thermoform aligners. Various thermoform materials may be used, such as polyvinyl chloride, polyurethane, polyethylene terephthalate, and PET-G.
The recent increasing interest in the emerging 3D-printed aligner market highlights the importance of conducting thorough studies.[13] While direct 3D-printed aligners have the potential to eliminate a step in the aligner fabrication process, they also introduce various variable factors correlated to the material and the printing technology that can impact the final product.[14] Therefore, careful investigation and analysis are necessary to understand and optimize the outcomes of this technology.
Studies have examined the print accuracy and thickness of 3D-printed orthodontic appliances, yielding varying results. Previous research has generally agreed that the accuracy of 3D-printed orthodontic appliances is comparable to thermoforming, if not better.[14-16] However, in terms of thickness, a study by Edelmann et al. found that aligners 3D printed with dental LT clear were approximately 0.200 mm thicker than their intended digital design.[17] While it is crucial to take into account these factors, the overall effectiveness of the appliance will ultimately be determined by its mechanical properties.
Tera Hartz TC-85 (Graphy Inc., Seoul, South Korea) is the first aligner introduced in the field that can be directly printed using digital light processing (DLP) technology. However, there is a lack of knowledge about its material properties.[18] DLP is a 3D printing technology that uses a digital light projector to cure liquid photopolymer resin layer by layer to create 3D objects. In DLP printing, the digital light projector displays a complete layer of the object onto the liquid resin, causing it to solidify or cure. By projecting an image of an entire layer at once, the curing process is expedited as all points within the layer are cured simultaneously.[19]
On the other hand, selective laser sintering (SLS) is a 3D printing technology that uses a high-power laser to selectively fuse or sinter powdered materials, typically plastic or metal, layer by layer to create 3D objects. In SLS printing, a laser scans and fuses the powdered material particles together based on a digital model, solidifying them to form the desired shape. Polyamide, a commonly used material in SLS 3D printing, exhibits reliable mechanical and thermal properties. However, there are limitations in utilizing this technology for polyamide due to incomplete information regarding the use of different types of additives and the impact of printing orientations on the material properties.[20].
Ideally, clear aligners should exert consistent light forces to facilitate the physiological movement of teeth. However, achieving this ideal scenario in reality can be challenging. Despite aligners being subjected to intermittent loads, it is important to take into account the phenomenon of stress relaxation, which is the most important property to be considered when evaluating aligners’ efficacy.[19] The reason is that this phenomenon can cause the force exerted by the aligner to decrease over time and such reduction can potentially impact the efficiency of tooth movement. It depends on several factors, including the applied load, temperature, mechanical properties of the aligner material, and its geometry. Quantifying this decay is of utmost importance to accurately predict the effectiveness of tooth movement and compare it to the desired outcomes.
The study aimed to assess the mechanical properties, with a specific focus on stress relaxation, of two different types of clear aligners: Zendura FLX (Bay Materials LLC, Fremont, California, USA), a single-layer thermoformed aligner made of Polyurethane (TPU), and Duran (SCHEU, Iserlohn, Germany), a single-layer aligner made of PET-G-modified. In addition, the study also examined direct 3D-printed aligners using two different printing technologies: Tera Hartz TC-85 (Graphy Inc. Seoul, South Korea) and Polyamide (Noxi, Sweden and Martina, Due Carrare, Padova, Italy). The investigation was conducted immersed in water and at a constant temperature of 37°C, to simulate the oral cavity environment. The null hypothesis asserts that there are no statistically significant differences in the stress release property among the four analyzed materials and within the observation period in the same specimen.
MATERIAL AND METHODS
For the present study, two thermoplastic aligner materials and two printed aligners were chosen [Table 1]. The Zendura FLX (Bay Materials LLC, Fremont, California, USA) and Duran (SCHEU, Iserlohn, Germany) were thermoformed from single-layer disks of 0.76 mm thickness, respectively, made of polyurethane (TPU) and PET-G -modified.
Product name | Manufacturer | Composition | Thickness (mm) | |
---|---|---|---|---|
Thermoformed aligner | Zendura FLX | Bay materials LLC, Fremont, California, USA | Polyurethane (TPU) | 0.76 |
Thermoformed aligner | Duran | Scheu-dental, Iserlohn, Germany | PET-G-modified | 0.76 |
3D-printed aligner | Tera | Graphy Inc, Seoul, South Korea | Tera Harz TC-85 | 0.75 |
3D-printed aligner | Noxi | Sweden-Martina, Due Carrare, Padova, Italy | Polyamide | 0.75 |
TPU: Thermoplastic polyurethanes, PET-G-modified: Polyethylene terephthalate glycol.
The Tera Hartz TC-85 (Graphy Inc., Seoul, South Korea) was 3D printed directly using the SprintRay Pro S (SprintRay Inc., Los Angeles, California 90065, USA) with DLP Refined. It was then cured using Tera Harz Cure (Graphy Inc., Seoul, South Korea). The Polyamide (Noxi, Sweden and Martina, Due Carrare, Padova, Italy) was directly 3D printed using the SLS plastic printing technology on an EOS 3D Printer (EOS GmbH, Krailling, Germany). The design and thickness of 0.7 mm for each of the two printed aligners was created on computer-aided design (CAD).
Before testing, the thickness was measured and confirmed using a digital external measuring gauge (Kroeplin K110, Kroeplin GmbH, Gartenstrabe 50, 36381 Schluchtern, Germany). The gauge is characterized by a measurement range of 0–10 mm and a resolution of 0.001 mm.
The same upper model of a previously orthodontically treated patient was chosen to thermoform the first two products of clear aligners and then to design and 3D-print the other two samples of the study.
A motorized vertical testing bench TVO-S (AstraLab, Mariano Comense, Italy) was used to conduct a one-point controlled and vertical compression to apply a constant preset load [Figure 1].
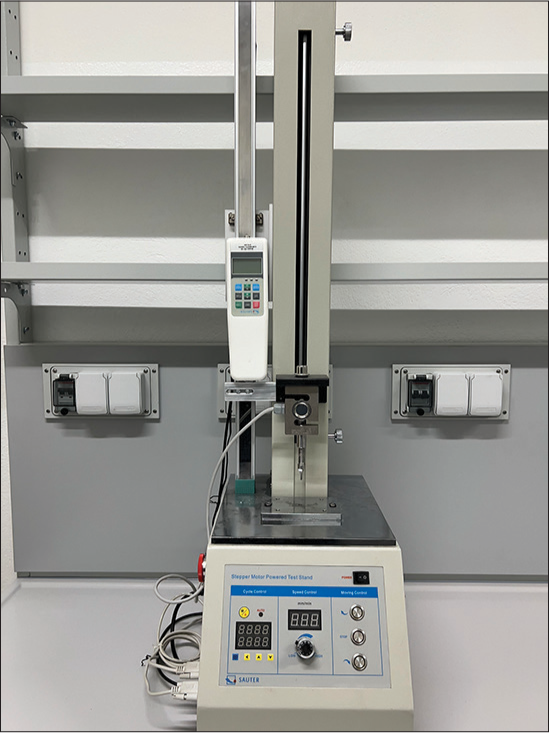
- Motorized vertical testing bench TVO-S (AstraLab, Mariano Comense, Italy).
The 3D-printed stereolithography model was affixed to a horizontal support using Superglue, in order to have the force application always in the same point and perpendicular to the plane. Then, each aligner sample was positioned on the model to perform the test.
The upper right central incisor (1.1) was extracted from the model, near the location where force would later be applied to the aligner, without any cuts being made. The horizontal stand was positioned in a bath measuring 20 cm × 20 cm × 10 cm, filled with distilled water at 37°C. The bath was positioned under the load cell. To keep the water temperature at 37°C, a Julabo Labortechnik GmbH immersion heater (Seelbach, Germany) was placed in a separate water bath filled with distilled water as well. Both water baths were connected with inlet and outlet pipes [Figure 2].
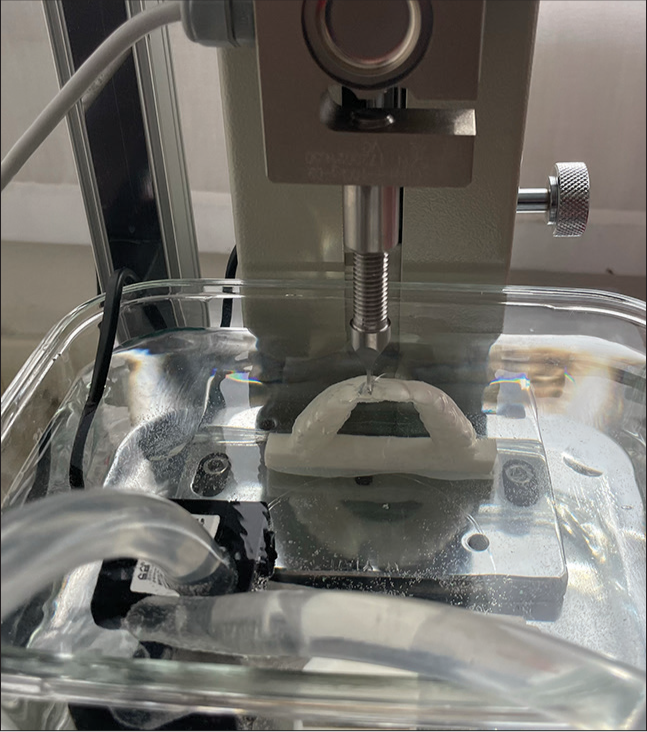
- The aligner sample immersed in the bath and positioned under the load cell.
A stress-relaxation test was performed for each sample for 8 consecutive h. The length of the testing period was chosen as it represents the maximum consecutive time that the aligner is typically worn in the oral cavity during normal usage. Moreover, a recent study examined the behavior of clear aligners over a 24-h period, and found that the majority of stress relaxation occurred during the initial 8 h under a constant load. Subsequently, from 16 to 24 h, the percentage of stress relaxation reached a stable plateau with minimal variations.[21]
After the aligner was correctly positioned on the model, 1 mm/min of velocity was set,and the deformation of 0.5 mm was achieved within the initial 30 ss of the test. As the upper right central incisor was previously removed from the model, the magnitude of deflection of the aligner at that point could be measured because there is no contact with the model. It simulates a clinically maximal activation condition and, therefore, deflection of the aligner when delivered to the patient. A recent systematic review with meta-analysis defines the staging of treatment with aligners, with an activation ranging between 0.2 mm and 0.5 mm.[22] This range of activation has also been confirmed by more recent in vitro studies, made on different materials, determined by thin-film pressure sensors.[23-25]
The deflection load of 0.5 mm remained constant throughout the observation period, during which data were collected every 1 s, in order to have a precise stress-relaxation curve for each material. For convenient reading of the measured value, a support for force gauges with external measuring cell on test benches, Sauter TVO-A01, was connected to the computer where the data transmission software, Sauter AFH Fast (AstraLab, Mariano Comense, Italy), was installed. A stress relaxation curve, force (N)/time (s), was then provided by the machine for each observation period [Figure 3].
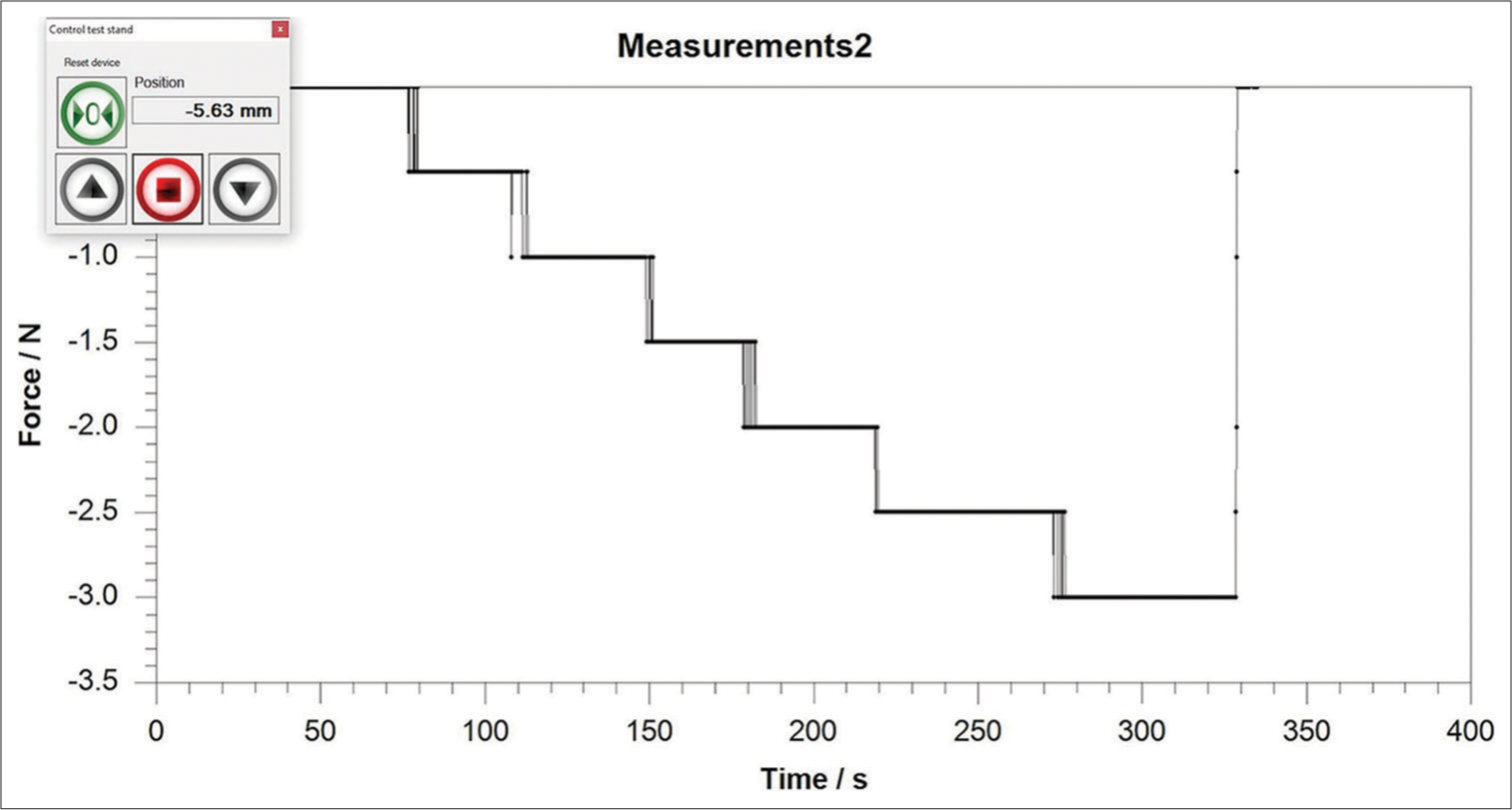
- Transmission software, Sauter AFH Fast (AstraLab, Mariano Comense, Italy). A screenshot as an example of the software interface, taken at an intermediate point during the observation period. period, N: Newton.
Two equivalent tests were conducted on the same specimen one consecutive to the other (test 1 and test 2), with 8-h relapse, to simulate the intermittence of deflection force, to establish, and compare the mean initial and final stress for each material. Moreover, to compare the stress degradation of each sample, the normalized stress, represented by the following equation, was employed to calculate the percentage of stress decay:
Stress decay % = σ/σmax × 100
where σ represents the initial stress of the material achieved within the initial 30 s of the test and σmax the maximum stress reached in the observation period. The decay percentage was calculated after 1 h, 2 h, and 8 h.
Statistical analysis
A paired-sample t-test was performed to compare, for each material, whether there was a significant difference between the mean stress of the first test and the mean stress of the second at 1 h, 2 h, and 8 h. The differences from a statistical point of view were verified after 1 h, 2 h, and 8 h. The specific test used analysis of variance (ANOVA) or Brown-Forsythe test) depends on the result of the Levene test. If the ANOVA or Brown-Forsythe test shows a statistical significance, pairwise comparisons are conducted.
To compare the stress relaxation measurements of each material, the non-parametric Wilcoxon test was employed due to the insufficient sample size for a parametric test. Furthermore, the stress relaxation after 8 h among the materials was assessed using the Kruskal–Wallis nonparametric test, followed by pairwise comparisons. The statistical analyses were conducted with an alpha significance level of 0.05. IBM Statistical Package for the Social Sciences Statistics version 28 software was utilized for data analysis.
RESULTS
For both Test 1 and Test 2, a total of 28,800 measurements were recorded for each aligner, with one measurement taken per second over a duration of 8 h/test. These measurements were utilized to construct two stress relaxation curves based on the mean values for each aligner and visually compare all the samples tested [Figures 4 and 5].
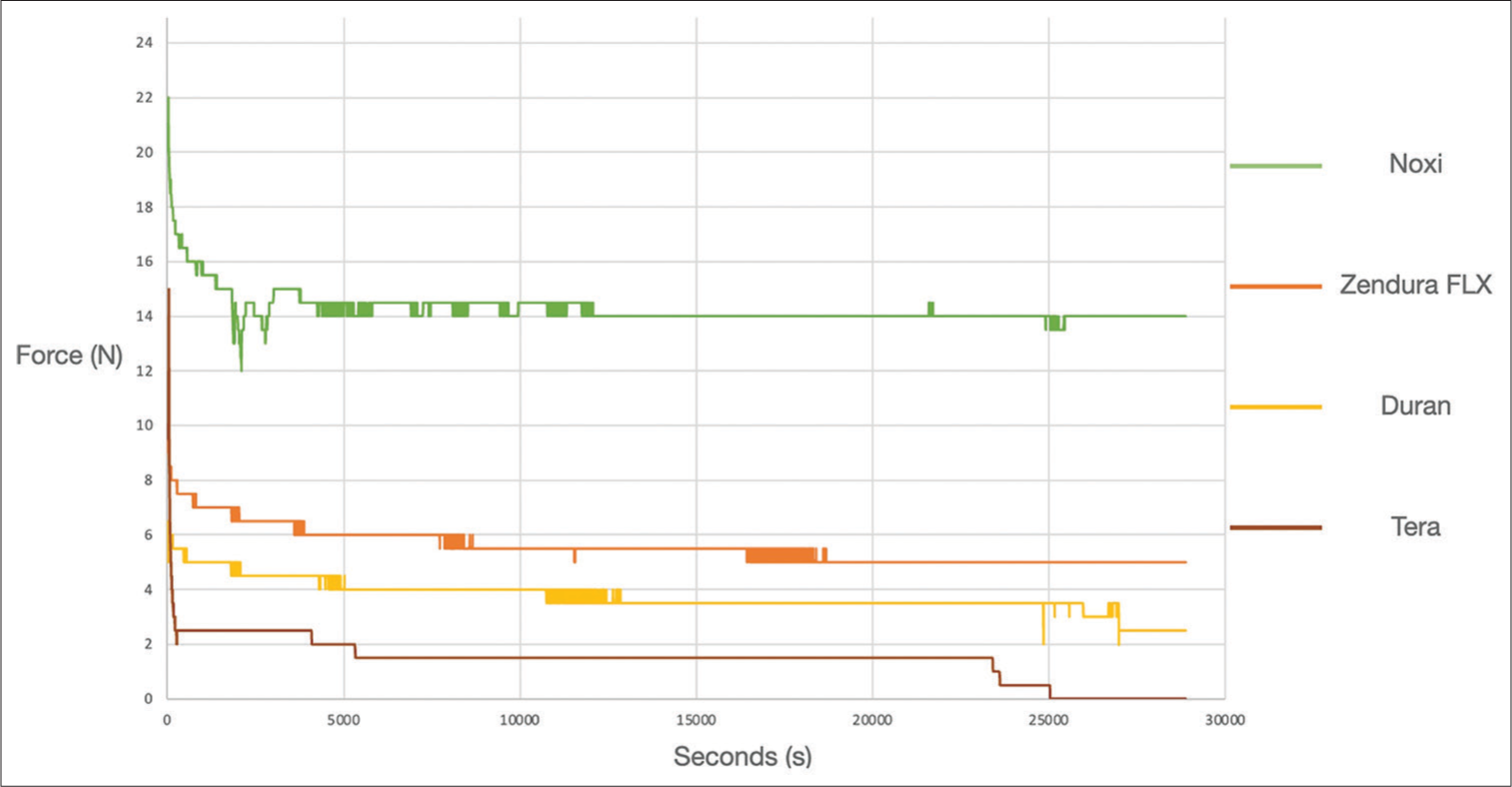
- Test 1, Stress relaxation curves of the four materials analyzed, Noxi: Polyamide, N: Newton.
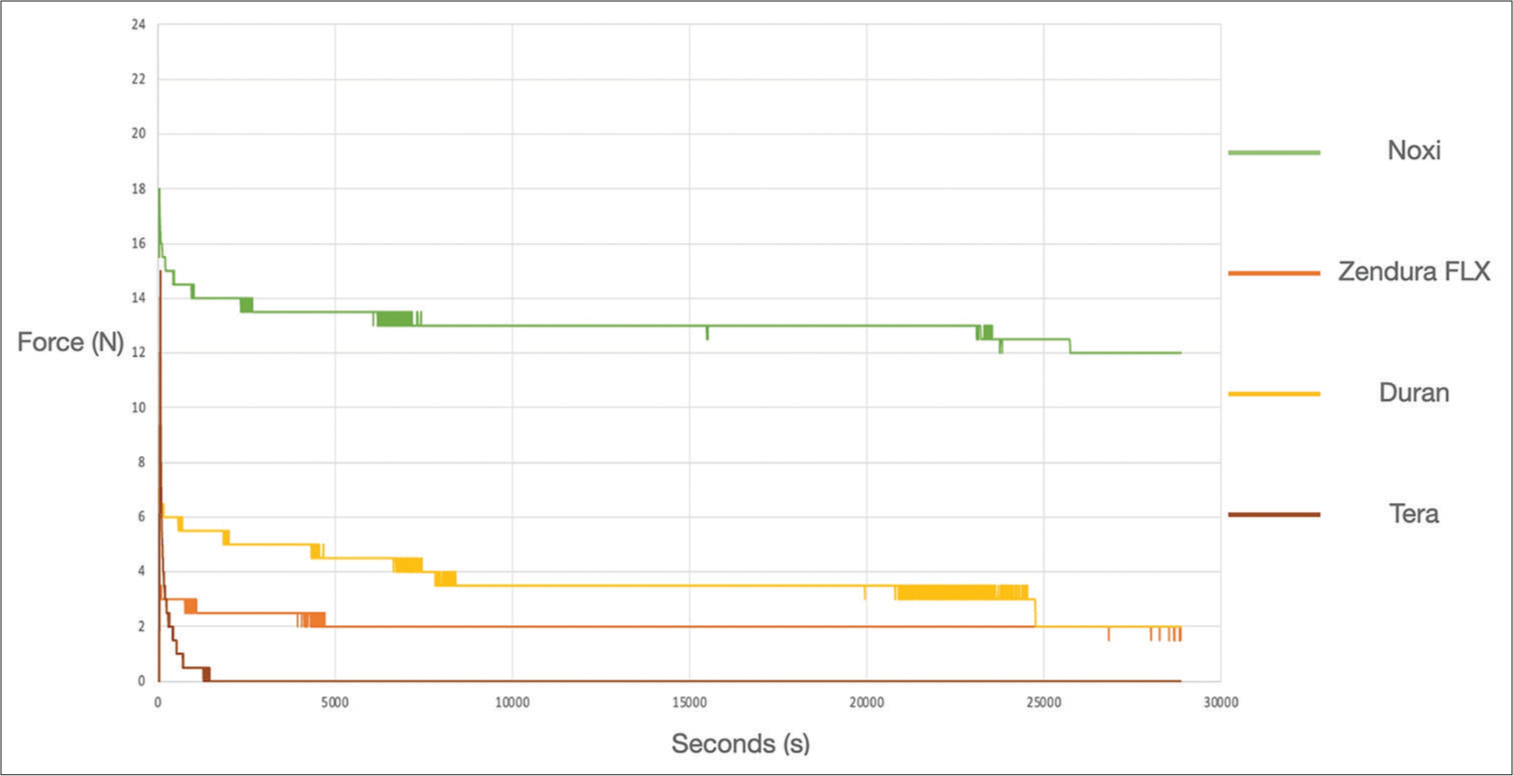
- Test 2, conducted after an 8-h period of relapse: Stress relaxation curves of the four materials analyzed, Noxi: Polyamide, N: Newton.
From all data collected, a mean initial and final stress value, and consequent mean stress decay percentage for each of the four aligners were identified [Table 2].
Material | Test 1 | Test 2 | ||||
---|---|---|---|---|---|---|
Initial force (N) | Final force (N) | Stress decay (%) | Initial force (N) | Final force (N) | Stress decay (%) | |
Zendura FLX | 9.5 | 5 | 47.4 | 3.5 | 2.5 | 29 |
Duran | 6.5 | 2.5 | 62 | 7 | 5 | 29 |
Tera | 14.5 | 0 | 90 | 13.5 | 0 | 100 |
Noxi | 20.5 | 14 | 32 | 17.5 | 13.5 | 23 |
N: Newton.
In both tests, Noxi showed the greatest stress value during the all 8 h period, with the most reduced stress decay percentage. Although Tera material exhibited higher initial stress values compared to Duran and Zendura FLX, the decay percentage reached 90% in test 1 and 100% in test 2. Indeed, despite Zendura FLX and Duran showing lower initial stress values, their percentage of decay has allowed them to maintain higher final force values. These two materials, in particular, presented the most similar values to each other.
The paired-sample t-test was conducted to statistically compare the mean values observed for the same aligner after 1 h, 2 h, and 8 h of the first and second constant load. A statistically significant difference (P < 0.001) was found for all materials and at all time periods considered [Table 3]. This indicates that each material experiences a significant decline in mechanical properties from Test 1 to Test 2.
Time | Material | Mean test 1 (n) | Mean test 2 (n) | Test statistic | df | P-value |
---|---|---|---|---|---|---|
1 h | Zendura FLX | 6.9 | 2.6 | 765.2 | 3537.0 | <0.001 |
Duran | 4.8 | 5.4 | −232.7 | 3537.0 | <0.001 | |
Tera | 2.0 | −0.6 | 140.1 | 3537.0 | <0.001 | |
Noxi | 15.2 | 14.1 | 80.1 | 3537.0 | <0.001 | |
2 h | Zendura FLX | 6.5 | 2.4 | 1013.5 | 7105.0 | <0.001 |
Duran | 4.5 | 5.0 | −274.3 | 7105.0 | <0.001 | |
Tera | 1.9 | −0.3 | 203.4 | 7105.0 | <0.001 | |
Noxi | 14.8 | 13.8 | 139.7 | 7105.0 | <0.001 | |
8 h | Zendura FLX | 5.6 | 2.1 | 1237.5 | 28589.0 | <0.001 |
Duran | 3.7 | 3.6 | 41.0 | 28589.0 | <0.001 | |
Tera | 1.3 | −0.1 | 292.1 | 28589.0 | <0.001 | |
Noxi | 14.3 | 13.0 | 451.0 | 28589.0 | <0.001 |
df: Degree of freedom. P<0.05 statistically significant, Noxi: Polyamide.
The same test was applied to compare the overall percentage of decay for each aligner between Test 1 and Test 2. It was found to be statistically and significantly different for all aligners, except for Zendura FLX [Table 4]. Specifically, Tera shows a higher percentage of decay in Test 2 compared to Test 1. On the other hand, Noxi exhibits a significantly higher percentage of decay in Test 1 compared to Test 2.
Material | Test 1 median stress relaxation (%) |
Test 2 median stress relaxation (%) |
P-value |
---|---|---|---|
Zendura FLX | 47.4 | 29 | >0.9 |
Duran | 62 | 29 | 0.026 |
Tera | 90 | 100 | 0.020 |
Noxi | 32 | 23 | 0.010 |
P<0.05 statistically significant
The Levene test yielded a significant result (P < 0.001) for each time period, indicating that the assumption of homoscedasticity is not met. Indeed, to determine if there was a significant difference in terms of force among the four materials, a Brown-Forsythe test was conducted for each of the 3 time periods: after 1 h, after 2 h, and after 8 h. Significant differences were observed among the materials at all time periods (P < 0.001) [Table 5]. If the percentage of stress relaxation is considered, a significant difference among the four aligners was found as well (P < 0.001) [Table 4]. Furthermore, post hoc comparisons revealed significant differences among all pairs of materials (P < 0.001), except for stress relaxation (%) between Zendura FLX and Duran.
Time | Material | Mean test 1 (n) | Test statistic | Df1 | Df2 | P-value |
---|---|---|---|---|---|---|
1 h | Zendura FLX | 6.9 | 76175.0 | 3.0 | 6774.6 | <0.001 |
Duran | 4.8 | |||||
Tera | 2.0 | |||||
Noxi | 15.2 | |||||
2 h | Zendura FLX | 6.5 | 245428.7 | 3.0 | 17045.7 | <0.001 |
Duran | 4.5 | |||||
Tera | 1.9 | |||||
Noxi | 14.8 | |||||
8 h | Zendura FLX | 5.6 | 1793146.3 | 3.0 | 95984.1 | <0.001 |
Duran | 3.7 | |||||
Tera | 1.3 | |||||
Noxi | 14.3 |
df: Degree of freedom, P<0.05 statistically significant, Noxi: Polyamide
The null hypothesis was then rejected in all its parts.
DISCUSSION
The objective of the present in vitro study is to evaluate the stress relaxation properties of 3D-printed aligners in comparison to thermoformed aligners made from various materials.
As confirmed by previous studies, aligners exhibit varying mechanical properties based on the different characteristics of the materials and the different thicknesses employed. The choice to consider only one sample for each selected aligner in the current pilot in vitro study can be attributed to the previous studies where it has been observed that the difference in measurements between samples is practically negligible or clinically irrelevant.[21,26,27] Moreover, the same sample was used to conduct two repeated tests in order to simulate the condition of wear.
To recreate the condition of oral cavity, a 0.7 mm thickness aligner was completely immersed in a humid environment at 37°C and subjected to a constant load for 8 h.
The deflection magnitude was selected to replicate the aligner’s maximum deflection throughout the entire treatment duration. According to a recent systematic review incorporating meta-analysis, the treatment staging for aligners is defined with an activation range of 0.2–0.5 mm.[22]
If we consider the staging of movement, the magnitude of aligner activation is usually lower. However, given that aligner treatment exhibits a movement predictability ranging from 40% to 70% depending on the planned movement type, it is reasonable to assume that the magnitude of deflection increases as the aligner sequence progresses.[26-28] This is why, in this in vitro study, we decided to consider a higher degree of activation to simulate a more extreme clinical condition that occurs during the intermediate stages of treatment.
A recent study examined the behavior of clear aligners over a 24-h period and found that the majority of stress relaxation occurred during the initial 8 h under a constant load. Subsequently, from 16 h to 24 h, the percentage of stress relaxation reached a stable plateau with minimal variations.[25]
The initial stress decay is a characteristic feature of polymer stress relaxation. Ideally, aligners should exert a gentle and consistent force over-time.[29] However, the material should also be sufficiently rigid and have a high yield strength to ensure the force remains within the elastic range. This should be reflected by a horizontal flat curve, indicating a constant force that is adequate for tooth movements over time.
As expected, in the present study, the percentage of stress relaxation was found to be significant for all tested aligners, ranging from 23% to 100%, depending on the material and construction characteristics. In particular, in the present study, thermoformed aligner is compared to 3-direct-printed aligners.
The higher the percentage, the more concerns arise regarding the material’s capacity to withstand adequate forces for orthodontic tooth movement.
Within the oral cavity, aligners experience intermittent loads both in the short term (when the patient inserts them) and in the long term (due to continuous contact with the tooth being moved, which resists the movement). Stress relaxation occurs, reducing the load exerted by the aligner even when there is a constant deflection, before the tooth starts to move. The degree of reduction in load and its impact on performance will be influenced by both the applied load magnitude and the material properties of the aligner. Therefore, it is crucial to quantify this decay to predict the aligner’s ability to effectively move the teeth.
In an ideal scenario, an aligner should consistently apply a gentle and constant force. To achieve this, the ideal material should have a relatively flat relaxation curve, indicating its capability to maintain a constant and continuous force over time.
From this point of view, the four materials tested exhibited different characteristics, with possible different clinical implications. In general, the higher the median stress decay, the more force is lost for tooth movement.
As long as thermoformed aligners are compared, the previous findings revealed that the single-layer materials exhibited the highest values in terms of both absolute stress and rate of stress decay. In contrast, the double-layer materials demonstrated consistent stress release but 4 times lower than those observed in the single-layer samples.[30] However, this study considered only two single-layer thermoformed aligners, but made from two different materials: Zendura FLX from a polyurethane disc and Duran from a PET-G disc.
These two materials, in particular, presented the most similar values to each other. What is evident is an opposite behavior in the two tests: In Test 1, FLX exhibits higher values, while in Test 2, lower values are compared to Duran. This indicates that the first material was more affected by undergoing two consecutive stress tests.
If, on one hand, in test 2, Duran returns to high initial values similar to those in test 1, while FLX remains stable at much lower values, confirming that the performance of clear aligners should be investigated not only at the first insertion but also over time and after consequent activations. These results are partly consistent with previous studies. For instance, Zhang et al.[31] observed a rapid decline in stress within the initial 60 min of applying the load, similar to the findings of Fang et al.[32] who conducted tests over a 180-min period on various analyzed samples. However, the stress-release values reported by Fang et al.,[32] ranging from 33.5% to 50% of the initial stress, were similar to the values measured for thermoformed aligners in the present study. In a study by Lombardo et al.,[25] thermoplastic samples were tested for a duration of 24 h. The results showed that the rate of stress decay was highest during the initial 8 h and gradually approached a plateau during the remaining 16 h. Four samples were tested, and after 24 h, residual stress levels varied between 38% and 82%.
The percentages of stress relaxation observed in Zendura FLX and Duran aligners are generally higher in this study compared to previous studies. However, direct comparison with the current study is not possible because the previous studies evaluated the materials before the thermoforming phase, which could potentially alter the mechanical properties of the material. Furthermore, the lower values are certainly related to the use of reduced material thicknesses, specifically 0.7 mm in the current tests compared to 1/1.2 mm in the previous studies.[21,30]
What is novel in the present study is that thermoformed aligners, which have already been extensively investigated in the literature, were compared with two 3D-printed aligners using different printers and materials. Since the recent emergence of 3D printing and in-house fabrication methods for orthodontic appliances, a multitude of concerns have surfaced regarding various aspects. These concerns encompass uncertainties surrounding the physical and mechanical properties of the materials, chemical stability, broader biological profile, as well as technical aspects of the printing process itself.[17,33]
As long as the in vivo-aging is considered, Can et al.[34] suggested that the mechanical properties of the tested 3D-printed material did not deteriorate during the 1-week duration of intraoral usage.
Stress relaxation in directly printed aligners was investigated by Lee et al.,[35] and it was found to occur at a higher level compared to thermoformed PET-g aligners at 37°C.
More recently, a previous study analyzed the stress relaxation of various 3D-printed aligners, considering two different materials, one of which is not yet available commercially: Material X (Envisiontec, Inc; Dearborn, Mich) and OD-Clear TF (3DResyns, Barcelona, Spain).[32]
After a 2-h test, the residual stress of the wet samples at a 2% strain was <10% for both 3D-printed aligners, indicating a significant decrease in stress from the initial values. The authors also hypothesize that conducting a longer stress relaxation test would have resulted in even lower residual stress values.[32]
On the contrary, in the current test, significant differences were observed in the percentage of stress relaxation between the two printed aligners. Tera Hartz TC-85 (Graphy Inc. Korea) exhibited the lowest values, with a decay percentage that ranged from 90% in test 1–100% in test 2. On the other hand, Noxi maintained higher force levels compared to thermoformed aligners, thanks to low decay percentages in both test 1 (32%) and test 2 (23%). The dissimilar results compared to previous studies could be attributed to differences in the printer, resin, and the methodology employed to test the mechanical properties.
Moreover, although we attempted to simulate the first two days of usage by repeating the test twice, a future investigation should take into account the material fatigue that occurs after the aligner has been used for several days.
There are a few more considerations to acknowledge. First, it is essential to recognize that the performance of the aligner materials may exhibit variations during the actual wearing period in the oral cavity, which differs from the controlled in vitro conditions utilized in the simulations. Consequently, conducting further research, including a comprehensive finite element study, would be valuable in determining the aligner materials that are most suitable for the planned dental movements. This additional investigation would also provide deeper insights into the performance characteristics of these materials.
CONCLUSION
All the tested materials displayed substantial stress decay during the 8-h period of constant load, although significant differences were observed among the various materials under investigation.
The stress decay results in test 2 were lower compared to those evaluated in test 1 for all aligners analyzed, except for Tera. It is worth mentioning that all aligners began with a lower force value in test 2, except for Duran.
The Noxi printed aligner demonstrated the highest force values throughout the analyzed period and in both tests, confirmed by low percentages of stress relaxation ranging from 23% to 32%.
Additional studies are necessary to investigate the real-world behavior of orthodontic aligners during the course of treatment, particularly after in vivo aging.
Ethical approval
The study was conducted in accordance with the Declaration of Helsinki, and the protocol was approved by the Ethics Committee of University of Ferrara with the approval number 14/2022 (Ethical approval date: 9th May 2022).
Declaration of patient consent
Patient’s consent was not required as there are no patients in this study.
Conflicts of interest
Luca Lombardo is on the Editorial Board of the Journal.
Use of artificial intelligence (AI)-assisted technology for manuscript preparation
The authors confirm that there was no use of artificial intelligence (AI)-assisted technology for assisting in the writing or editing of the manuscript and no images were manipulated using AI.
Financial support and sponsorship
Nil.
References
- Adult patients' adjustability to orthodontic appliances. Part I: A comparison between Labial, Lingual, and Invisalign™. Eur J Orthod. 2012;34:724-30.
- [CrossRef] [PubMed] [Google Scholar]
- Short-term chemical and physical changes in Invisalign appliances. Aust Orthod J. 2009;25:34-40.
- [CrossRef] [PubMed] [Google Scholar]
- Characterization of TPU-elastomers by thermal analysis (DSC) Polym Test. 2004;23:413-7.
- [CrossRef] [Google Scholar]
- Comparing the compatibility of various functionalized polypropylenes with thermoplastic polyurethane (TPU) Polymer. 2004;45:1981-91.
- [CrossRef] [Google Scholar]
- Efficacy of various multilayers of orthodontic clear aligners: A simulated study. Comput Methods Biomech Biomed Engin. 2022;25:1710-21.
- [CrossRef] [PubMed] [Google Scholar]
- Effectiveness of clear aligner therapy for orthodontic treatment: A systematic review. Orthod Craniofac Res. 2020;23:133-42.
- [CrossRef] [PubMed] [Google Scholar]
- Advances in orthodontic clear aligner materials. Bioact Mater. 2022;22:384-403.
- [CrossRef] [PubMed] [Google Scholar]
- Outcome assessment of orthodontic clear aligner vs fixed appliance treatment in a teenage population with mild malocclusions. Angle Orthod. 2020;90:485-90.
- [CrossRef] [PubMed] [Google Scholar]
- Treatment effectiveness of young adults using clear aligners versus buccal fixed appliances in class I malocclusion with first premolar extraction using the ABO-Objective Grading System: A randomized controlled clinical trial. Int Orthod. 2023;21:100817.
- [CrossRef] [PubMed] [Google Scholar]
- Assessment of the occlusal outcomes in patients treated with orthognathic surgery and clear aligners. Orthod Craniofac Res. 2023;26:371-7.
- [CrossRef] [PubMed] [Google Scholar]
- Mechanical properties of thermoplastic polymers for aligner manufacturing: In vitro study. Dent J (Basel). 2020;8:47.
- [CrossRef] [PubMed] [Google Scholar]
- Effects of mechanical properties of thermoplastic materials on the initial force of thermoplastic appliances. Angle Orthod. 2013;83:476-83.
- [CrossRef] [PubMed] [Google Scholar]
- 3D printing of clear orthodontic aligners: Where we are and where we are going. Materials (Basel). 2020;13:5204.
- [CrossRef] [PubMed] [Google Scholar]
- Comparison of dimensional accuracy between direct-printed and thermoformed aligners. Korean J Orthod. 2022;52:249-57.
- [CrossRef] [PubMed] [Google Scholar]
- Evaluation of fit for 3D-printed retainers compared with thermoform retainers. Am J Orthod Dentofacial Orthop. 2019;155:592-9.
- [CrossRef] [PubMed] [Google Scholar]
- Mechanical and geometric properties of thermoformed and 3D printed clear dental aligners. Am J Orthod Dentofacial Orthop. 2019;156:694-701.
- [CrossRef] [PubMed] [Google Scholar]
- Analysis of the thickness of 3-dimensional-printed orthodontic aligners. Am J Orthod Dentofacial Orthop. 2020;158:e91-8.
- [CrossRef] [PubMed] [Google Scholar]
- Comparative analysis of mechanical properties of orthodontic aligners produced by different contemporary 3D printers. Orthod Craniofac Res. 2022;25:336-41.
- [CrossRef] [PubMed] [Google Scholar]
- Comparison in terms of accuracy between DLP and LCD printing technology for dental model printing. Dent J (Basel). 2022;10:181.
- [CrossRef] [PubMed] [Google Scholar]
- Effect of additives and print orientation on the properties of laser sintering-printed polyamide 12 components. Polymers (Basel). 2022;14:1172.
- [CrossRef] [PubMed] [Google Scholar]
- Stress relaxation properties of five orthodontic aligner materials: A 14-day in-vitro study. Bioengineering (Basel). 2022;9:349.
- [CrossRef] [PubMed] [Google Scholar]
- Forces and moments generated by aligner-type appliances for orthodontic tooth movement: A systematic review and meta-analysis. Orthod Craniofac Res. 2019;22:248-58.
- [CrossRef] [PubMed] [Google Scholar]
- Effects of time and clear aligner removal frequency on the force delivered by different polyethylene terephthalate glycol-modified materials determined with thin-film pressure sensors. Am J Orthod Dentofacial Orthop. 2019;155:98-107.
- [CrossRef] [PubMed] [Google Scholar]
- Experimental study of the pressures and points of application of the forces exerted between aligner and tooth. Nanomaterials (Basel). 2019;9:1010.
- [CrossRef] [PubMed] [Google Scholar]
- Stress relaxation properties of four orthodontic aligner materials: A 24-hour in vitro study. Angle Orthod. 2017;87:11-8.
- [CrossRef] [PubMed] [Google Scholar]
- Effect of clear aligner wear protocol on the efficacy of tooth movement. Angle Orthod. 2021;91:157-63.
- [CrossRef] [PubMed] [Google Scholar]
- Predictability of orthodontic movement with orthodontic aligners: A retrospective study. Prog Orthod. 2017;18:35.
- [CrossRef] [PubMed] [Google Scholar]
- Has Invisalign improved? A prospective follow-up study on the efficacy of tooth movement with Invisalign. Am J Orthod Dentofacial Orthop. 2020;158:420-5.
- [CrossRef] [PubMed] [Google Scholar]
- Effects of temperature changes and stress loading on the mechanical and shape memory properties of thermoplastic materials with different glass transition behaviours and crystal structures. Eur J Orthod. 2015;37:665-70.
- [CrossRef] [PubMed] [Google Scholar]
- Comparison of mechanical properties of 3-dimensional printed and thermoformed orthodontic aligners. Am J Orthod Dentofacial Orthop. 2023;163:720-8.
- [CrossRef] [PubMed] [Google Scholar]
- Preparation and characterization of thermoplastic materials for invisible orthodontics. Dent Mater J. 2011;30:954-9.
- [CrossRef] [PubMed] [Google Scholar]
- Dynamic stress relaxation of orthodontic thermoplastic materials in a simulated oral environment. Dent Mater J. 2013;32:946-51.
- [CrossRef] [PubMed] [Google Scholar]
- Effect of print orientation and duration of ultraviolet curing on the dimensional accuracy of a 3-dimensionally printed orthodontic clear aligner design. Am J Orthod Dentofacial Orthop. 2020;158:889-7.
- [CrossRef] [PubMed] [Google Scholar]
- In-house 3D-printed aligners: Effect of in vivo ageing on mechanical properties. Eur J Orthod. 2022;44:51-5.
- [CrossRef] [PubMed] [Google Scholar]
- Thermomechanical properties of 3D printed photocurable shape memory resin for clear aligners. Sci Rep. 2022;12:6246.
- [CrossRef] [PubMed] [Google Scholar]