Translate this page into:
Effect of the bracketless orthodontics technique and resin composite material on the biomechanical response of the upper central incisor: 3D finite element analysis
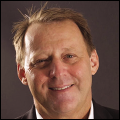
*Corresponding author: Guaracy Lyra Fonseca, Department of Orthodontics, Guaracy Fonseca, Boa viagem, Av. Boa viagem, 3804 ap 2701, Recife - 51021-000, Pernambuco, Brazil. guaracyorto@gmail.com
-
Received: ,
Accepted: ,
How to cite this article: Fonseca GL, Neto NT, do Lago Prieto MG, Azevedo F, Harrop C, Oliveira N. Effect of the bracketless orthodontics technique and resin composite material on the biomechanical response of the upper central incisor: 3D finite element analysis. APOS Trends Orthod 2022;12:34-43.
Abstract
Objectives:
The bracketless orthodontic treatment (BOT) is an alternative technique which indicates using an orthodontic appliance composed of wires and composite resin assisted by 3D technology. However, the biomechanical response of central incisor orthodontic movement has yet to be investigated. Thus, the aim of the present investigation was to calculate the stress magnitude in central incisor movement through 3D finite element analysis using different wire diameters (0.012”, 0.014”, and 0.016”) of nickel–titanium wire and two different resin composites (Opallis and Filtek).
Materials and Methods:
A 3D volume composed of enamel, dentin, cortical bone, cancellous bone, periodontal ligament, composite resin, and different orthodontic wire diameters was designed. After the modeling process, the models were exported to computer-aided engineering software divided into a finite number of elements, and a mechanical structural static analysis was conducted.
Results:
The stress results were plotted on colorimetric maps and in tables for comparison between the different models. The results showed that the central incisor orthodontic movement with BOT does not induce damage to the periodontal ligament, dental root, or bone tissue, regardless of the simulated orthodontic wire diameter and resin composite materials. The palatal composite resin and orthodontic wire also presented acceptable stress magnitude during orthodontic movement.
Conclusion:
Thus, the BOT technique promoted a suitable biomechanical response during central incisor movement regardless the resin composite.
Keywords
Biomechanics
Orthodontics
Finite element analysis
Tooth movement techniques
INTRODUCTION
It has been very common for patients to be more resistant to the use of orthodontic appliances with brackets, especially after childhood, even to ceramic brackets considered more esthetic than metal brackets. As an alternative, invisible aligners are an appropriate treatment option when correctly indicated in situations of controlled orthodontic movement.[1]
Lingual brackets are another esthetic option for orthodontic appliances as they are bonded to the lingual surface of the teeth. However, this technique has disadvantages such as poor hygiene, modified phonation, and tongue discomfort due to the volume of the device. In addition, aligners are not actually invisible or imperceptible and depend on the patient’s assiduous collaboration for proper use.[2] As the use of aligner systems requires a minimum use of 20 h a day, this therapeutic modality is directly associated with regularity and patient collaboration.[2-4]
Bracketless orthodontics treatment (BOT) it is an alternative concept of orthodontic appliance composed of fixed wires with direct composite resin.[3] This orthodontic treatment modality arose from the lack of stabilizing anterior teeth with fixed retainers, associated with the need for controlled movement and alignment. Thus, with BOT, it is possible to move the teeth in a controlled manner without metallic or ceramic brackets by pre-activating orthodontic wires.[2,3]
The previous studies state that teeth alignment by BOT should be used as the first treatment option when esthetics is considered essential for patient compliance because the appliance is not visible.[4] In addition, patients who have been treated with BOT have reported more comfort than lingual brackets.[4]
It is widely known that orthodontic tooth movements must be performed with nickel–titanium wires or multifilament wires following the biomechanical principles common to conventional orthodontic treatments and respecting the physiology of oral tissues.[2,4]
The tridimensional (3D)-BOT technology technique is advantageous for: (1) Controlling protrusion or lingualization by prior individualization of orthodontic wires in prototype models, (2) controlling the final shape of the arc and the desired movement, (3) controlling the necessary vertical and anteroposterior movements, (4) informing the orthodontist in advance which movements is required in the planning in degrees and millimeters, (5) enabling the previous construction of the arches, minimizing the office time, and increasing the patient’s confidence in the treatment through demonstrations through the physical model, helping the professional to establish the total treatment time with greater precision, (6) not interfering in the diction and phonetics of patients and with little interference in hygiene, (7) being an esthetic and comfortable device, (8) being a fast and efficient technique, (9) depending very little on the patient’s cooperation, as it is a fixed technique; and (10) being a self-ligating, straight wire, and play-free technique which allows the thread to slip when necessary.[1,5]
Despite the described advantages, evaluating the biomechanical response of the BOT technique in anterior teeth has not yet been evaluated in the literature. This evaluation is of interest to dentists since different diameters of orthodontic wire and types of composite resin can be used and thus modify the treatment success.
One of the bioengineering tools which can be applied to evaluate orthodontic movements is the finite element analysis (FEA) method. FEA is a reliable, economical, and fast computational numerical analysis. This research methodology can be applied to assist in treatment planning, understand bone remodeling, determine the center of resistance, and understand the biomechanics of tooth movement, thus contributing to advance orthodontics.[6-8]
Therefore, the objectives of this study were to evaluate (through 3D FEA): The stress distribution in an upper left incisor; the stress in the adhesive interface and in the orthodontic wire, as well as the microdeformation generated in the periodontal ligament and alveolar bone during the simulated movement using three different orthodontic wire thicknesses and two composite resin types in the BOT technique.
MATERIALS AND METHODS
A previously reported 3D maxillary model was considered for the present study.[8] The bone tissue was modeled with characteristics of periodontal health and absence of any pathological changes [Figure 1]. The model was then imported into computer-aided design software (Rhinoceros version 4.0 SR8; McNeel North America, Seattle, WA). The upper left central incisor (the study object) was then isolated through flat section cuts. The final geometries present in the 3D model were defined as cortical bone, medullary bone, periodontal ligament, dental root, enamel, and dental pulp.[9-11] Next, the models were verified as volumetric solids with an absence of defective surfaces through analysis of the edges used in the modeling protocol.
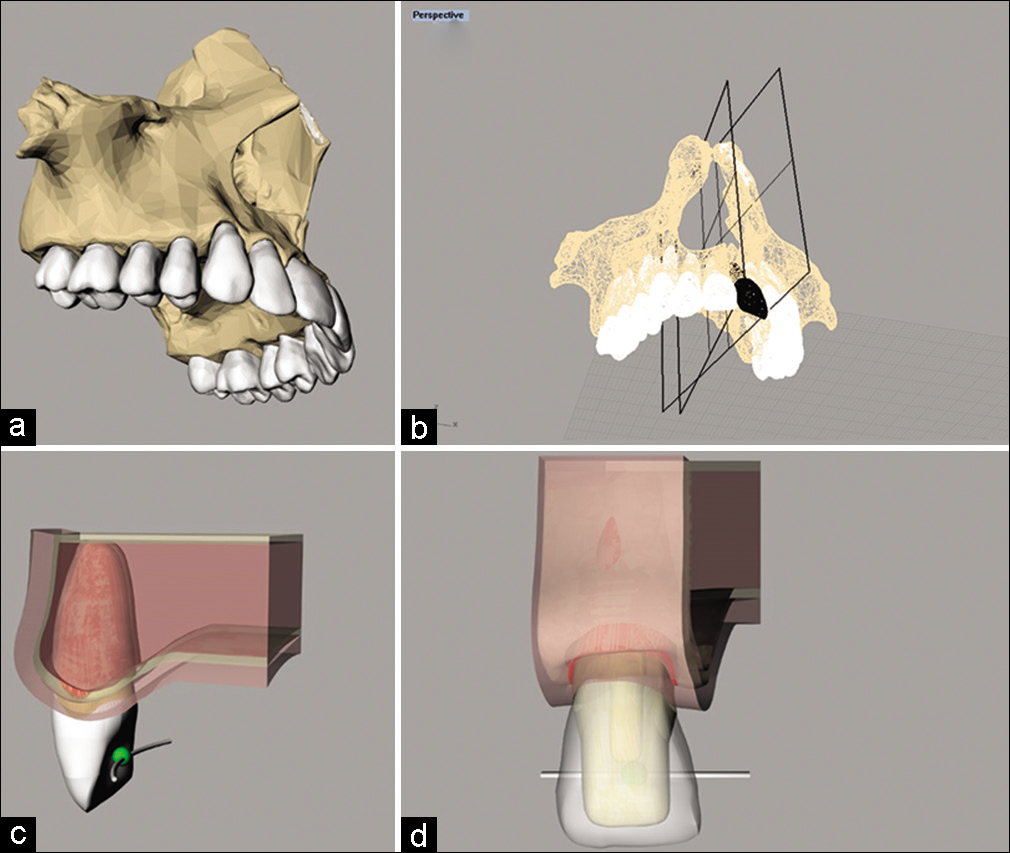
- Perspective view. (a) Three-dimensional model of dentate maxilla; (b) tooth study object selected to export to STL; (c and d) section of the maxillary bone with the 3D model containing a palatal resin bracket and orthodontic wire.
The model was created considering the composite resin technique to design the geometric structures of the resin brackets, respecting the minimum increments and the contact area with the palatal surface of an upper central incisor and ideal occlusion of the modeled dental element [Figure 2].
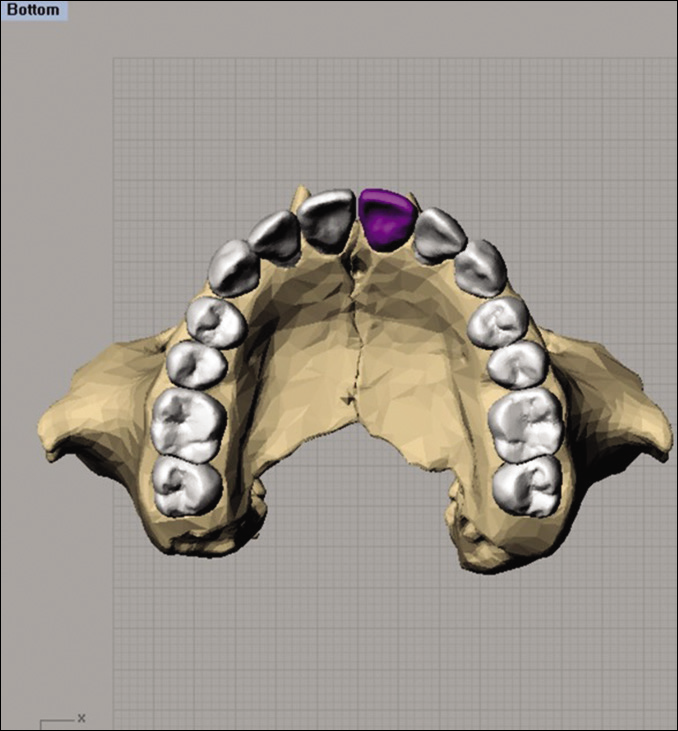
- Occlusal view of the 3D model based for representation of the spatial position of the orthodontic wire.
Next, the different diameters of orthodontic wires were created with a cylindrical, homogeneous, and uniform structure defined from a reference polyline, positioned inside the composite resin as clinically indicated [Figure 3]. Each finished model was exported to computer-assisted engineering software (ANSYS 19.2; ANSYS Inc., Houston, TX) in STEP (Standard for the Exchange of Product Data) format for mesh division and FEA [Figure 4].
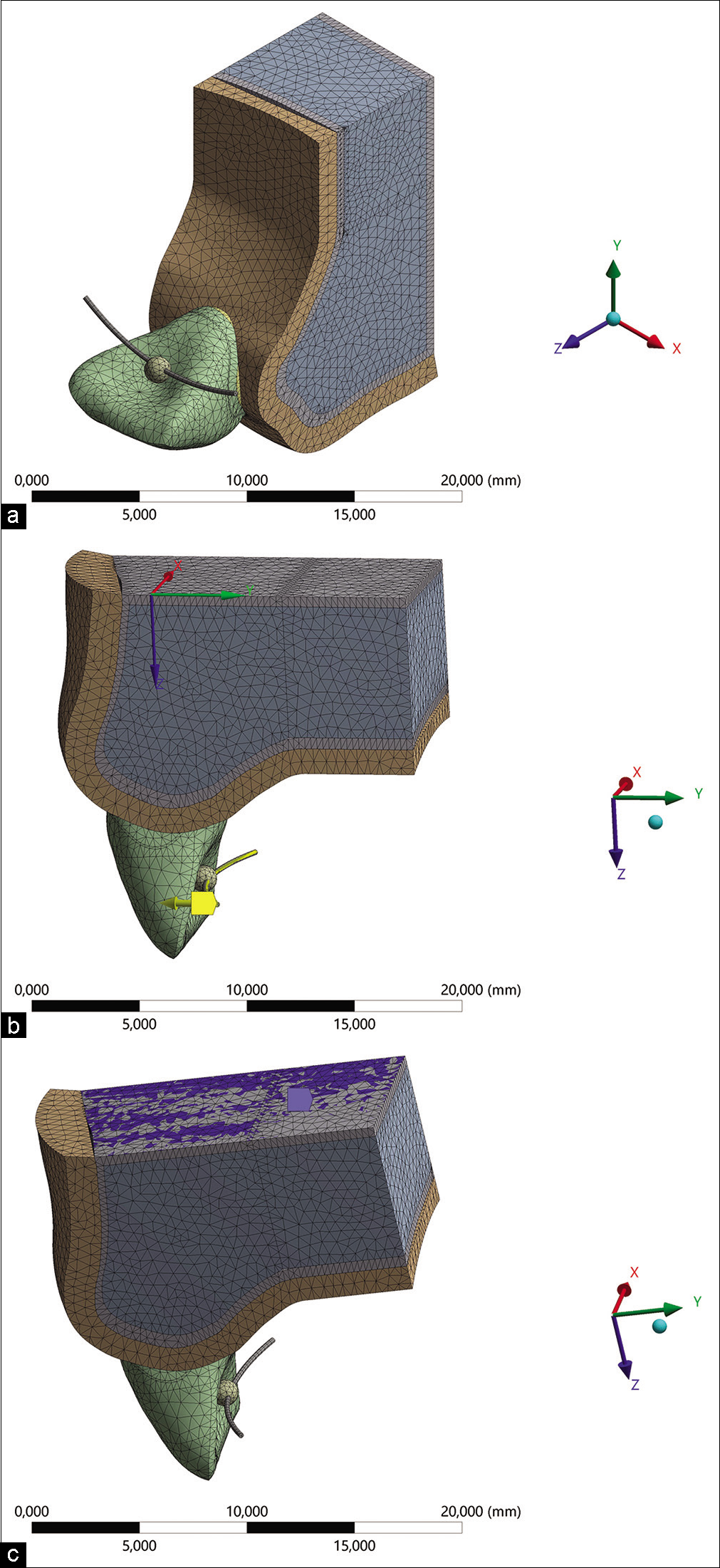
- (a) View of the mesh formed by the refined computer-aided engineering software after the convergence test with adequate definitions for the mechanical structural static analysis, (b) contour condition with activation direction indicated by the yellow arrow on the orthodontic wire, and (c) fixation of the cortical bone as support for the analysis.
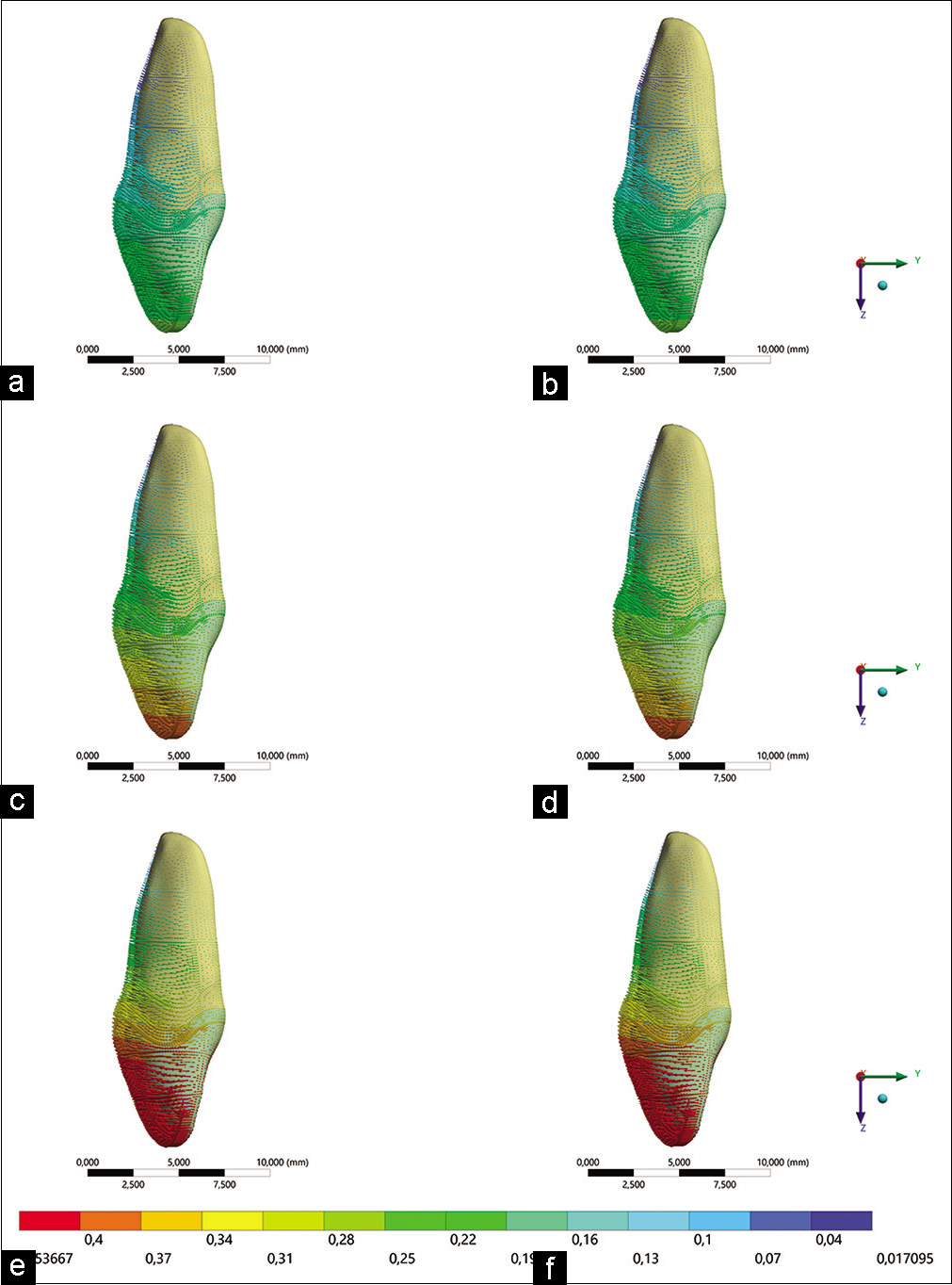
- Movement trend of models. (a) Filtek resin with 0.012 wire, (b) Opallis resin with 0.012 wire, (c) Filtek resin with 0.014 wire, (d) Opallis resin with 0.014 wire, (e) Filtek resin with 0.016 wire, (f) Opallis resin with 0.016 wire.
After exporting the 3D models, mechanical structural static analysis was performed simulating orthodontic movement for 1.0 mm of buccal displacement. Then, the mechanical properties (elastic modulus and Poisson’s coefficient) of each component simulated in the present study were defined based on the literature, assuming isotropic, homogeneous, and linearly elastic mechanical behavior [Table 1].
Material | Elastic modulus (MPa) |
Poisson ratio | References |
---|---|---|---|
Enamel | 84,100 | 0.30 | 9 |
Dentin | 18,600 | 0.30 | 9 |
Periodontal ligament | 50 | 0.45 | 9 |
Cortical bone | 13,700 | 0.33 | 9 |
Medullary bone | 1400 | 0.31 | 9 |
Filtek bulk fill resin posterior | 134,600 | 0.18 | 10 |
Opallis resin | 15,400 | 0.30 | 11 |
Orthodontic wire | 36,000 | 0.30 | 12 |
Then, the contacts were verified between each structure, being considered glued between the simulated human tissues, and frictional between orthodontic wire and the composite resin, allowing its sliding during movement. The system fixation was defined at the base of the maxilla bone tissue.
After calculating the mesh convergence with 10% linearity, the subdivision of the models into a finite number of nodes and elements was performed. The loading was based on displacing the orthodontic wire during controlled buccal movement of 1.0 mm.[12,13]
The required results were: The displacement tendency (total deformation) based on the fulcrum point of the tooth during orthodontic movement,[14] microdeformation in bone tissue,[15] minimum and maximum principal stress for the periodontal ligament,[16] minimum and maximum principal stress for the tooth root,[17] von Mises stress for orthodontic wire,[18] and maximum principal stress for the adhesive interface of the composite resin bracket.[19] In addition to the stress distribution maps, the maximum values of each analysis were plotted for quantitative comparison. The reaction force on the bone tissue was also calculated as a function of the load applied to the orthodontic wire.[20-22]
RESULTS
The analysis of the alveolar bone reaction force in the periodontal ligament of the central incisor comparatively quantified the load distributed over the alveolus [Table 2]. The analysis of tooth displacement to verify the trend of tooth movement in the different models is presented in [Figures 4 and 5]. It is observed that there is a similar vestibular movement trend between the models, but with greater vestibular displacement due to the increase in the diameter of the orthodontic wire applied, but with no difference for the composite resin type. The maximum movement values in mm are shown in [Table 3].
Composite resin | Orthodontic wire diameter (In) | Reaction force (Newtons) |
---|---|---|
Bulk fill | 0.012 | 0.83 |
0.014 | 1.27 | |
0.016 | 1.71 | |
Opallis | 0.012 | 0.82 |
0.014 | 1.25 | |
0.016 | 1.71 |
Composite resin | Orthodontic wire diameter (In) | Displacement |
---|---|---|
Bulk fill | 0.012 | 0.259 |
0.014 | 0.395 | |
0.016 | 0.538 | |
Opallis | 0.012 | 0.258 |
0.014 | 0.394 | |
0.016 | 0.536 |
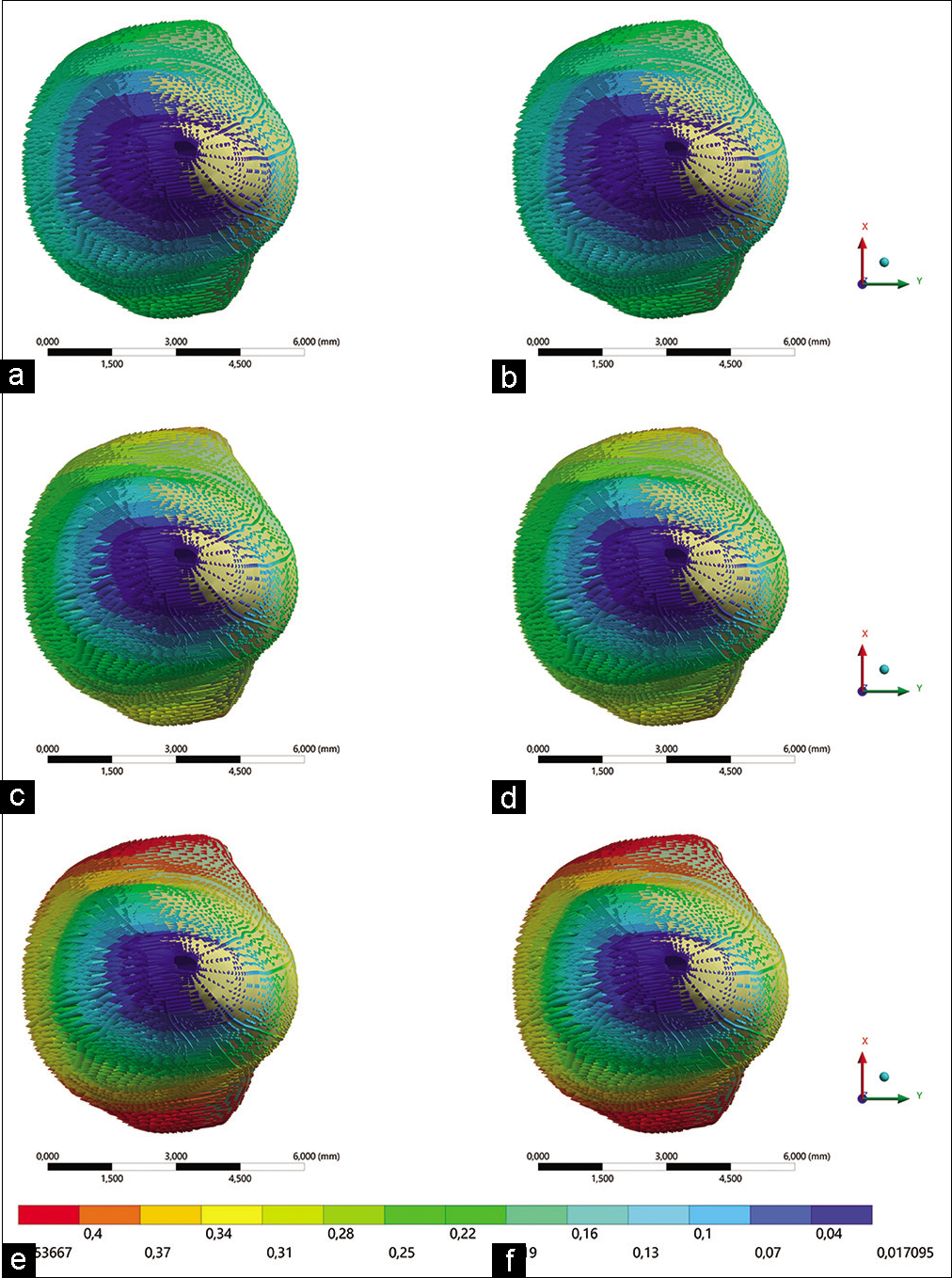
- Root portion trend of the movement of the models. The arrows indicate the tooth displacement direction and its intensity (red for greater displacement; green for less displacement). (a) Filtek resin with 0.012 wire, (b) Opallis resin with 0.012 wire, (c) Filtek resin with 0.014 wire, (d) Opallis resin with 0.014 wire, (e) Filtek resin with 0.016 wire, (f) Opallis resin with 0.016 wire.
An analysis of the periodontal ligament deformation over the alveolar bone evidences the comparison of the force variation impact on the trend of orthodontic movement in the simulated models. [Figure 6] shows the results for the periodontal ligament according to the strain deformation criterion; and [Figure 7] shows the results for the periodontal ligament according to the compression criterion. There is a notable greater compression magnitude for the palatal surface of the dental root, with values proportional to the diameter of the orthodontic wires and with no visible difference regarding the composite resin type. Peaks are shown in [Table 4] for quantitative comparison.
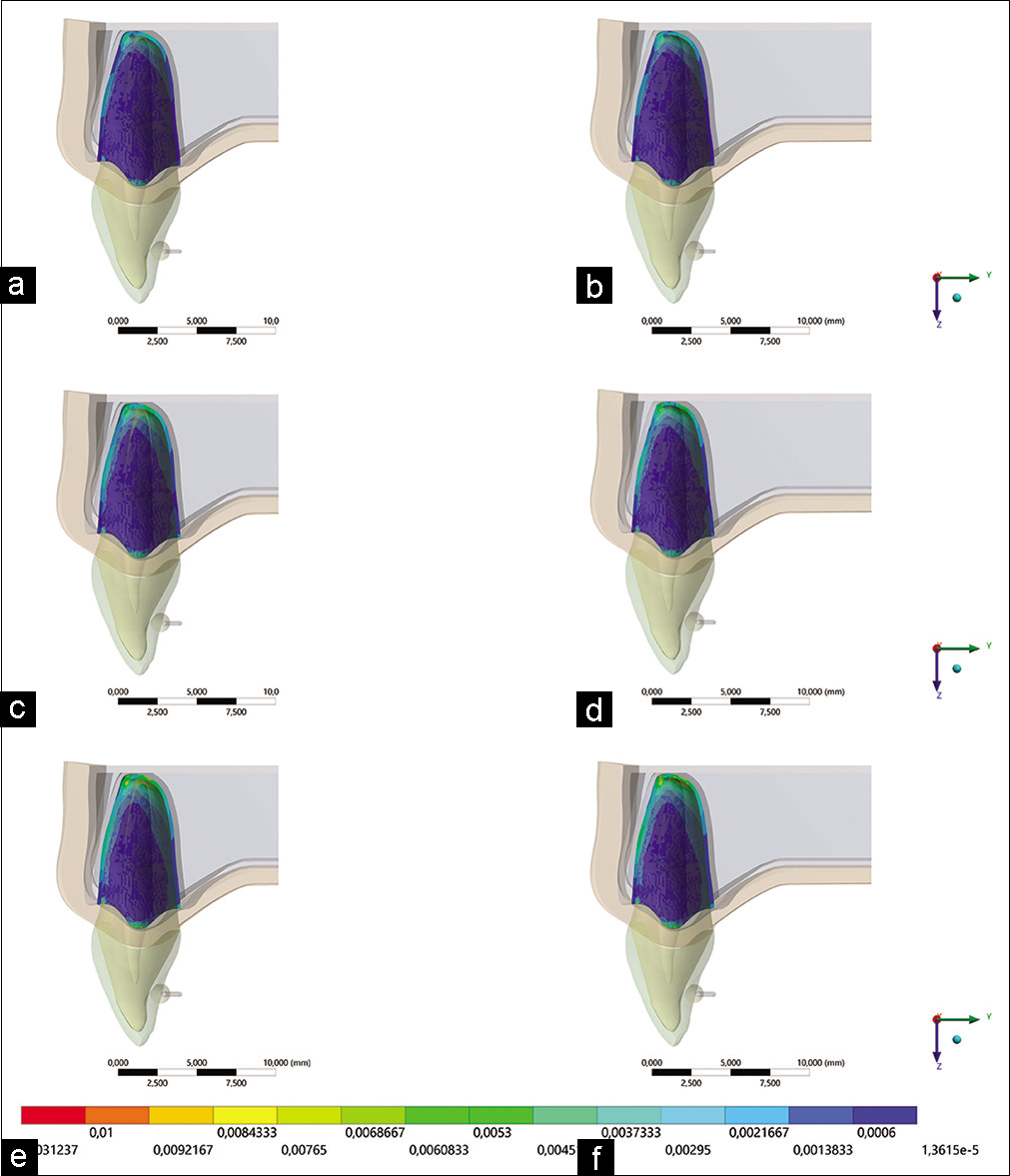
- Maximum principal deformation in the periodontal ligament. (a) Filtek resin with 0.012 wire, (b) Opallis resin with 0.012 wire, (c) Filtek resin with 0.014 wire, (d) Opallis resin with 0.014 wire, (e) Filtek resin with 0.016 wire, (f) Opallis resin with 0.016 wire.
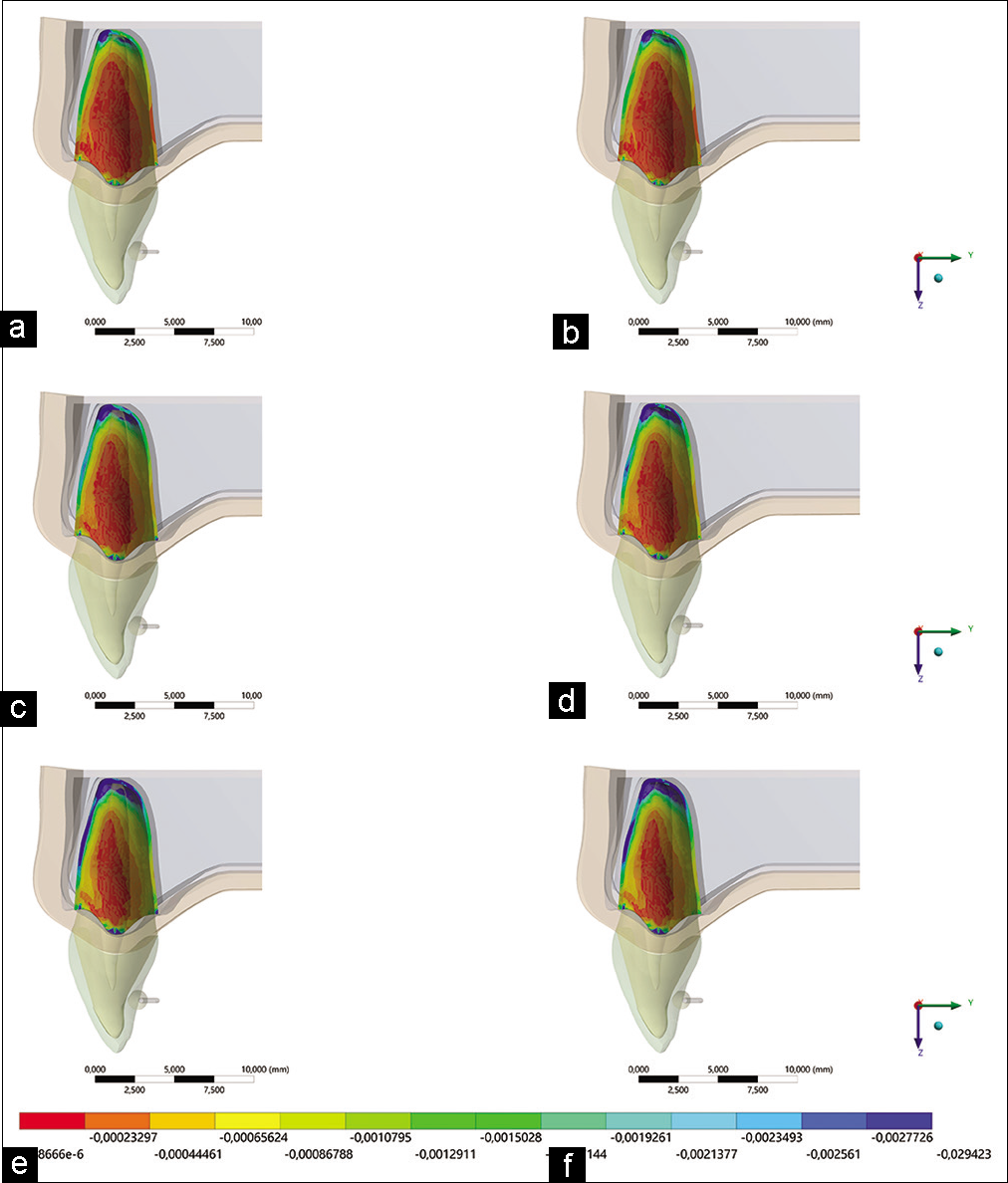
- Minimal principal deformation in the periodontal ligament. (a) Filtek resin with 0.012 wire, (b) Opallis resin with 0.012 wire, (c) Filtek resin with 0.014 wire, (d) Opallis resin with 0.014 wire, (e) Filtek resin with 0.016 wire, (f) Opallis resin with 0.016 wire.
Composite resin | Orthodontic wire diameter (In) | Compression | Tensile stress |
---|---|---|---|
Bulk fill | 0.012 | −0.0141 | 0.0150 |
0.014 | −0.0216 | 0.0229 | |
0.016 | −0.0294 | 0.0312 | |
Opallis | 0.012 | −0.0142 | 0.0151 |
0.014 | −0.0216 | 0.0230 | |
0.016 | −0.0294 | 0.0313 |
In the analysis of the alveolar bone tissue [Figure 8], an increase in the compression zones could be verified proportionally to the periodontal ligament deformation [Figure 9]. Assuming the limiting physiological values presented by Frost (1994) according to Wolff ’s law, values above 1500 με tend to activate lamellar bone remodeling, leading to reformulation and reinforcement, while values above 3000 με cause remodeling disorganization which generates irreversible microdamage to the bone. Thus, none of the models simulated in the present study present irreversible microdamage values to bone tissue [Table 5].
Composite resin | Orthodontic wire diameter (In) | Microdeformation |
---|---|---|
Bulk fill | 0.012 | 1143 |
0.014 | 1739 | |
0.016 | 2367 | |
Opallis | 0.012 | 1151 |
0.014 | 1743 | |
0.016 | 2373 |
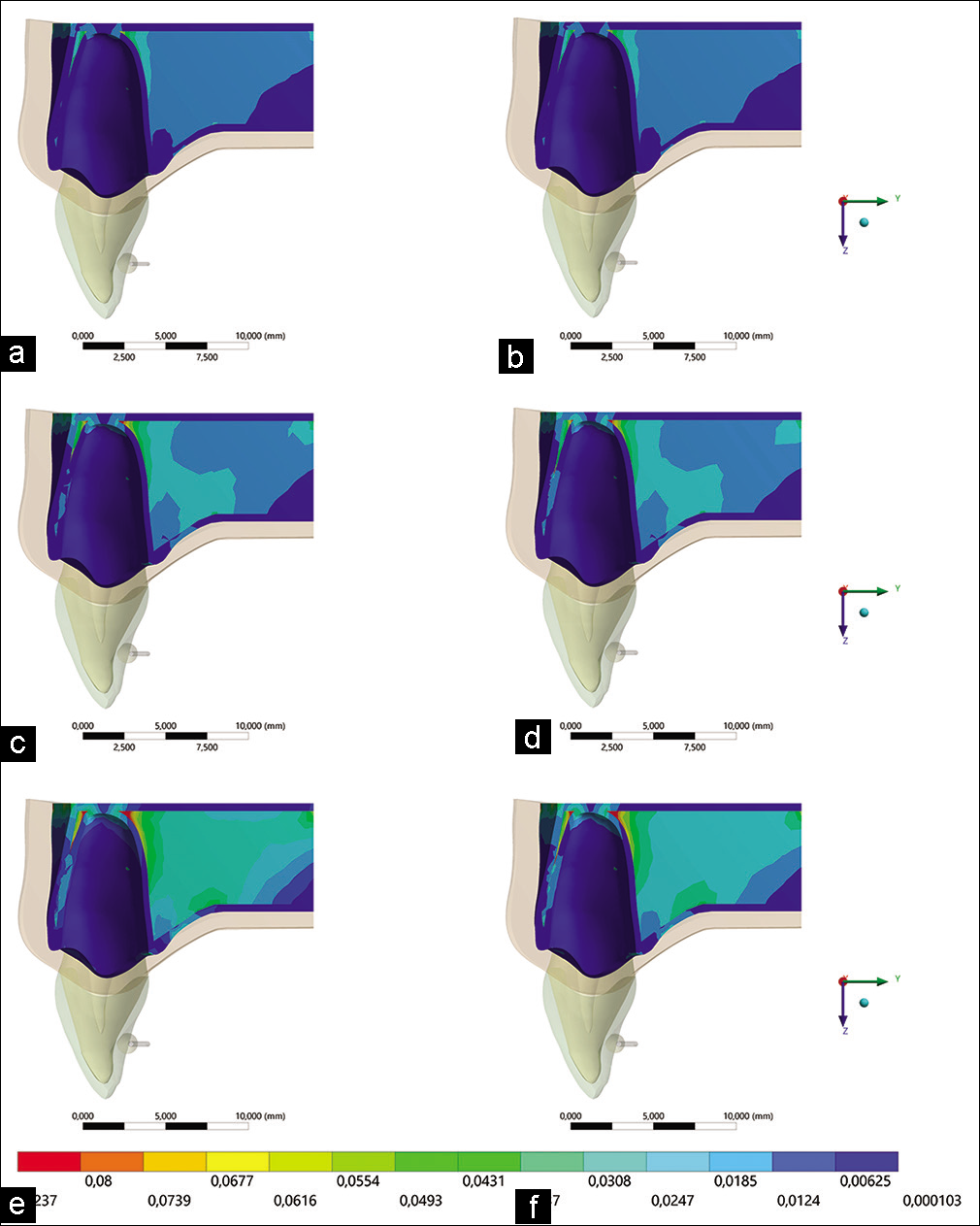
- Von Mises deformation in bone tissue. (a) Filtek resin with 0.012 wire, (b) Opallis resin with 0.012 wire, (c) Filtek resin with 0.014 wire, (d) Opallis resin with 0.014 wire, (e) Filtek resin with 0.016 wire, (f) Opallis resin with 0.016 wire.
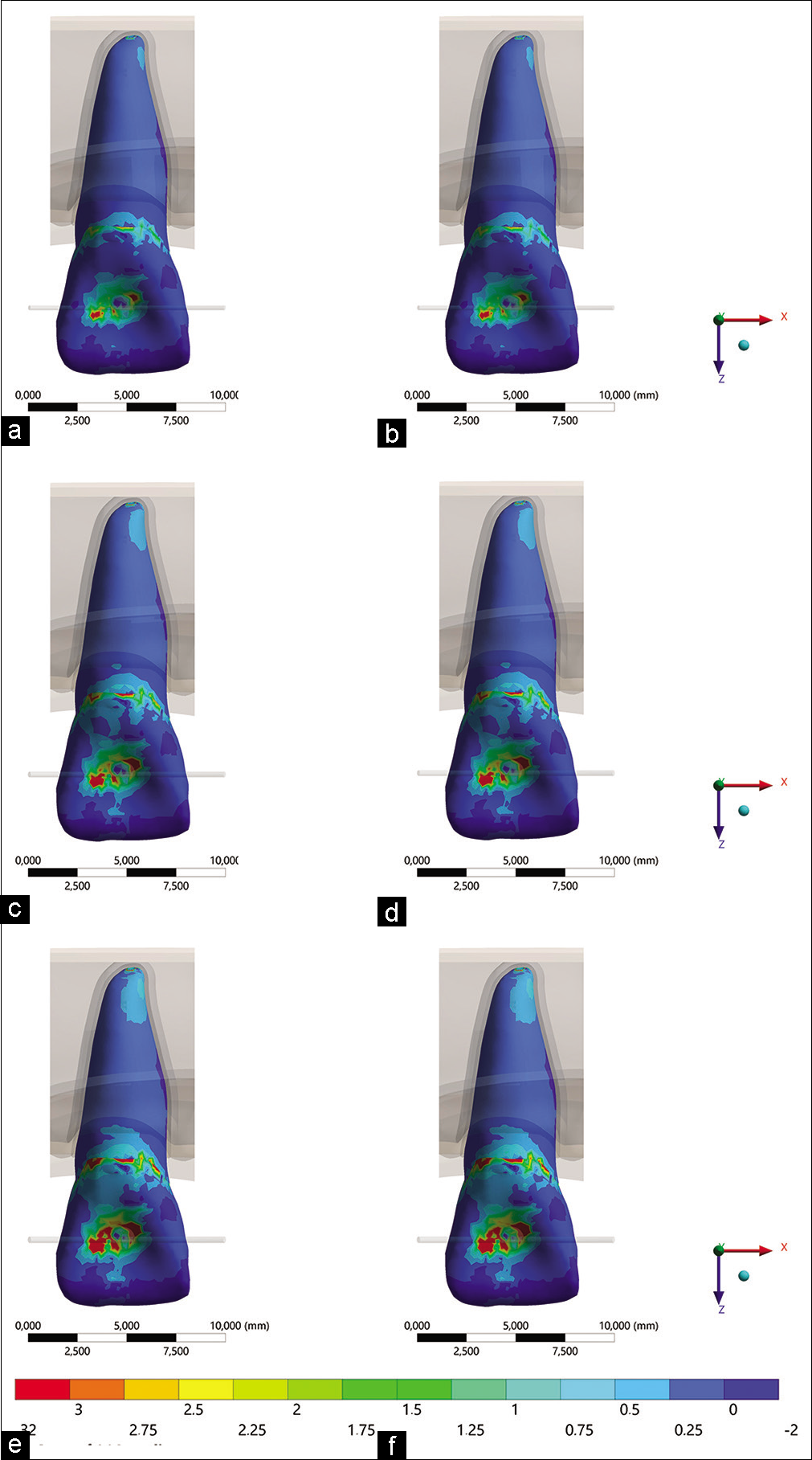
- Minimum principal stress in the dental root. (a) Filtek resin with 0.012 wire, (b) Opallis resin with 0.012 wire, (c) Filtek resin with 0.014 wire, (d) Opallis resin with 0.014 wire, (e) Filtek resin with 0.016 wire, (f) Opallis resin with 0.016 wire.
Next, an analysis of the root dentin pressure on the alveolar bone to compare the impact of bone variation on the orthodontic movement trend in the models was performed [Figures 9 and 10]. Tensile and compression peaks in the tooth structure are shown in [Table 6].
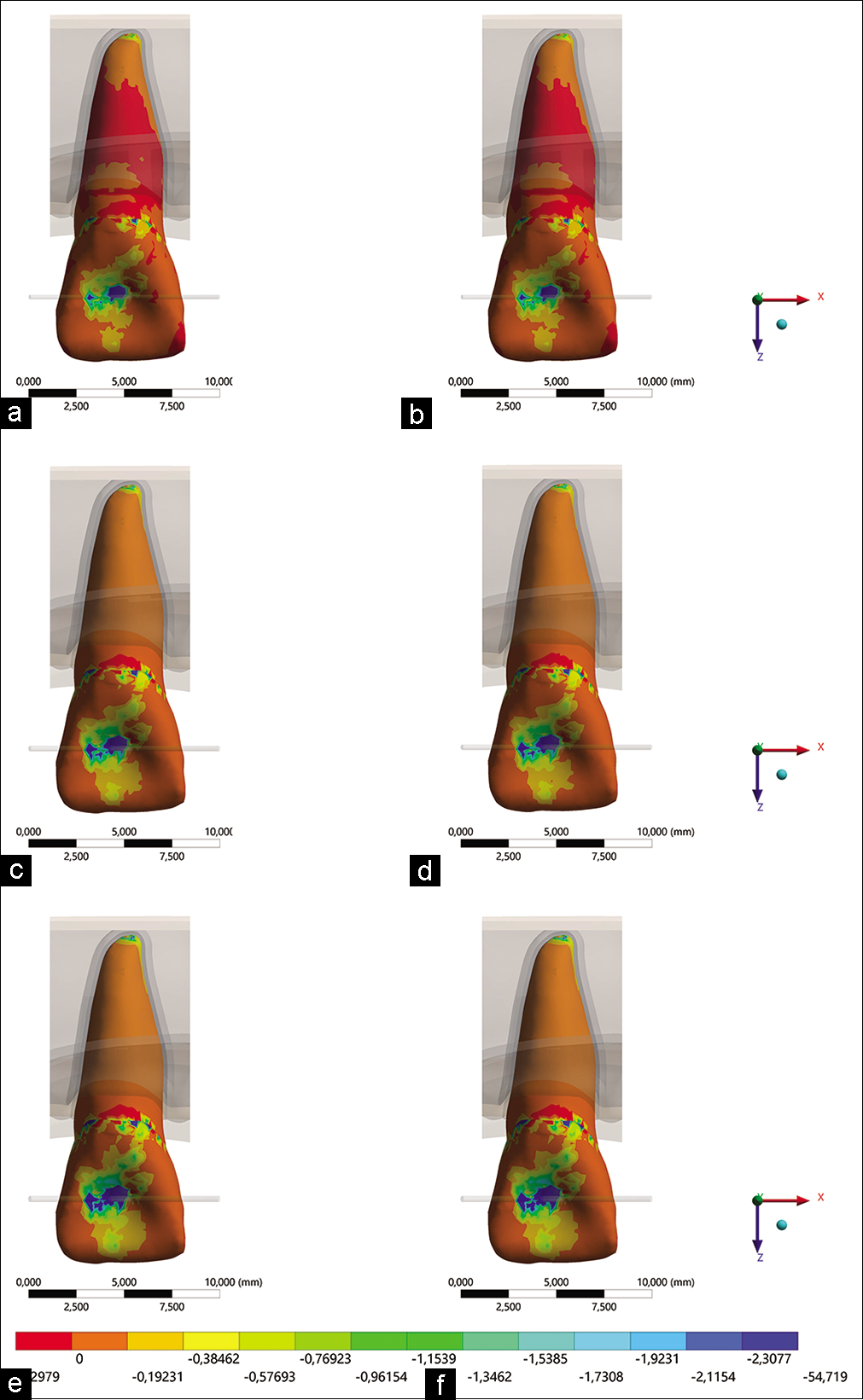
- Maximum principal stress in the dental root. (a) Filtek resin with 0.012 wire, (b) Opallis resin with 0.012 wire, (c) Filtek resin with 0.014 wire, (d) Opallis resin with 0.014 wire, (e) Filtek resin with 0.016 wire, (f) Opallis resin with 0.016 wire.
Composite resin | Orthodontic wire diameter (In) | Compression | Tensile stress |
---|---|---|---|
Bulk fill | 0.012 | −26.36 | 15.75 |
0.014 | −40.19 | 24.04 | |
0.016 | −54.71 | 32.69 | |
Opallis | 0.012 | −26.44 | 15.79 |
0.014 | −40.30 | 24.08 | |
0.016 | −54.87 | 32.78 |
To analyze the mechanical response, as well as the moment generated associated with the deformation of the orthodontic wire [Figure 11], the von Mises criterion was used.[23] However, none of the models presents plastic deformation during orthodontic activation applied during tooth movement based on the proportionality limit of the metal alloy of the orthodontic wire (close to 2000 MPa) [Table 7].
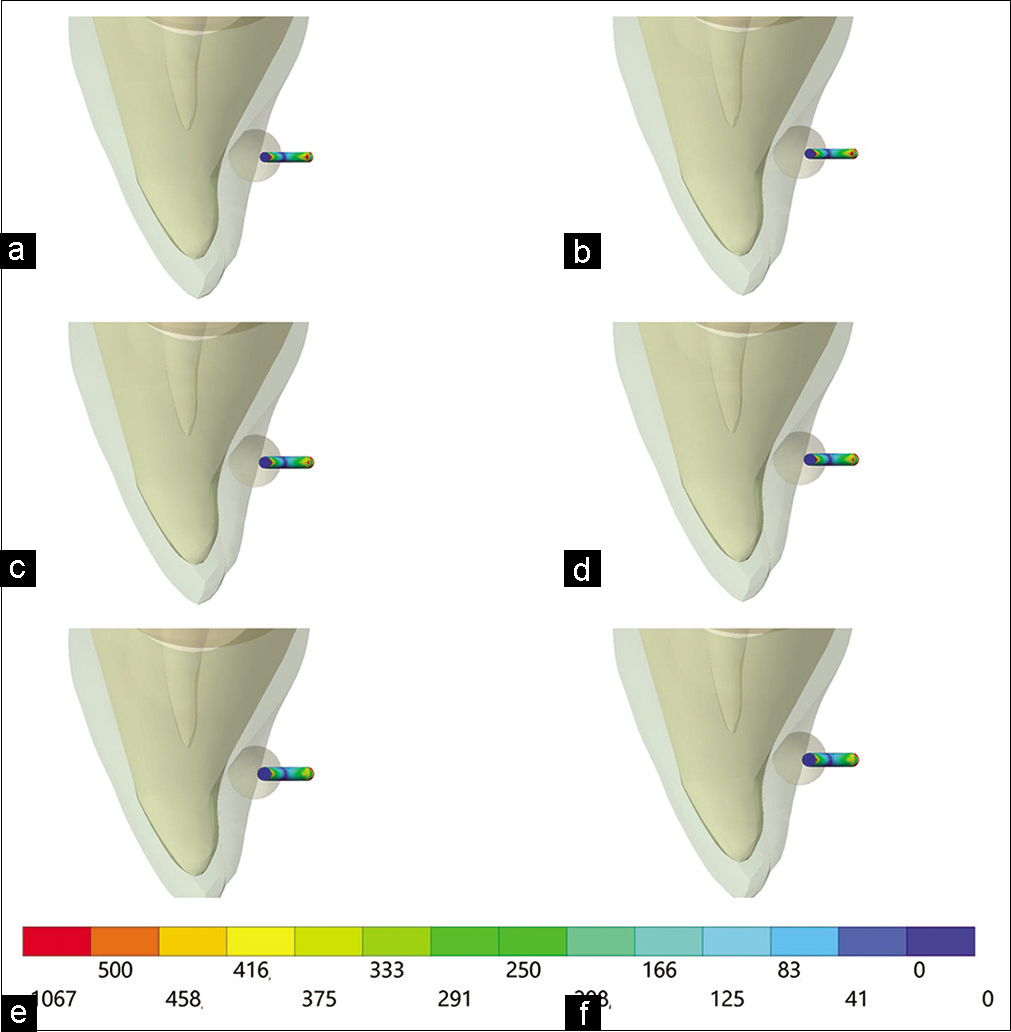
- Von Mises stress on orthodontic wire. (a) Filtek resin with 0.012 wire, (b) Opallis resin with 0.012 wire, (c) Filtek resin with 0.014 wire, (d) Opallis resin with 0.014 wire, (e) Filtek resin with 0.016 wire, (f) Opallis resin with 0.016 wire.
Composite resin | Orthodontic wire diameter (In) | Von Mises stress |
---|---|---|
Bulk fill | 0.012 | 1085 |
0.014 | 1067 | |
0.016 | 1040 | |
Opallis | 0.012 | 1086 |
0.014 | 1069 | |
0.016 | 1041 |
As the success of orthodontic treatment depends on keeping the wire in position for proper application of force, the composite resin was also investigated to assess the possibility of detachment. However, it is not possible to notice differences between the two resins types used in the present study [Figure 12], with decimal differences in peak values, which cannot be extrapolated as clinically significant [Table 8].
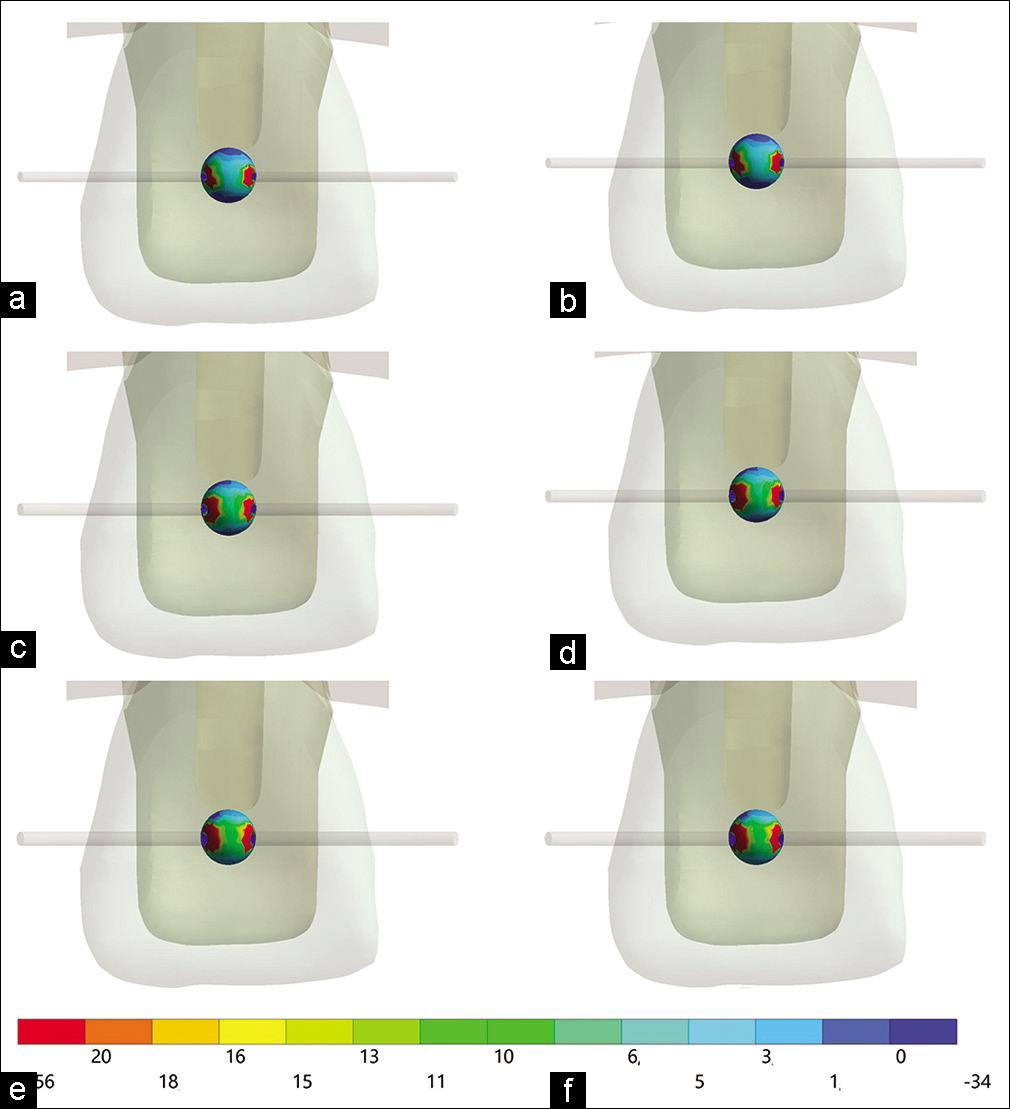
- Maximum principal stress in occlusal composite resin. (a) Filtek resin with 0.012 wire, (b) Opallis resin with 0.012 wire, (c) Filtek resin with 0.014 wire, (d) Opallis resin with 0.014 wire, (e) Filtek resin with 0.016 wire, (f) Opallis resin with 0.016 wire.
Composite resin | Orthodontic wire diameter (In) | Stress in the resin |
---|---|---|
Bulk fill | 0.012 | 21.96 |
0.014 | 17.06 | |
0.016 | 15.68 | |
Opallis | 0.012 | 23.90 |
0.014 | 18.61 | |
0.016 | 16.37 |
DISCUSSION
The correct use of alternative orthodontic techniques makes it possible to obtain appropriate results according to the desired movements in numerous cases. The present study demonstrated that the BOT technique promotes adequate stress and deformation values during tooth movement, regardless of the orthodontic wire diameter and the composite resin type used.
It is noteworthy that the BOT is an alternative technique to perform the desired tooth movement.[1,5] However, adequate fluid isolation, composite resin adhesion, and patient cooperation are necessary during the installation of the orthodontic appliance and during each device reactivation.[4]
As a disadvantage, BOT requires longer clinical consultation and requires occlusal lifting. However, this technique facilitates cleaning due to the absence of retentive orthodontic structures.
Understanding the stress distribution in the periodontium helps to predict the pain and potential damage which may occur even under functional bite force.[16] Thus, there will be a stimulus for bone tissue degeneration in situations of greater magnitude of compression stresses.[16] Basically, the mechanical response of the periodontal ligament initiates a cascade of biological events and induces the release of oxytocin which acts on alveolar bone remodeling; in addition, they can simultaneously make moved teeth susceptible to orthodontically induced inflammatory root resorption.[22] Nevertheless, none of the models simulated in the present study appear to be capable of generating root resorption with reaction forces <0.1 N in the medullary region of the bone and strain levels below 43 KPa.
The results obtained regarding the response generated in bone tissue did not exceed the maximum physiological limit to induce severe bone resorption.[15] On the contrary, models with 0.14 and 0.16 mm wire displacement tend to activate lamellar bone remodeling (with values >1500).
The von Mises criterion considers that the ductile material deformation starts when the concentrated stress magnitude vector reaches a critical value. This plasticity theory can be well applied to ductile materials, such as orthodontic wires with linear elastic behavior.[23] However, the stress value calculated in the present study suggests that there will be no damage to the orthodontic wire structure, regardless of the diameter and the composite resin used.
Numerous parameters are able to modify the adhesive strength and adhesion durability of the composite resin to the enamel, such as the homogeneity of the adhesive interface, the hydrophilicity of the adhesive surface, the dental substrate type, the adhesive characteristics, the composite resin strength, as well as its physicochemical properties.[24] Thus, the tensile stress represents one of the main results of strength in adhesive failure of dental restorations.[25] In this sense, the present study demonstrates that both simulated composite resins present similar mechanical response in all results evaluated. However, it is important to emphasize that different results can be observed in teeth with severe palatal wear and dentin exposure restored with direct and indirect biomaterials, with unbalanced parafunction and occlusion, which can affect the efficiency of this orthodontic treatment modality.
A previous study with FEA concluded that modifying the adhesive base of the bracket significantly influences the amount of stress generated at the adhesive interface. The authors suggest that more in-depth investigations are needed in other projects regarding stress in brackets, as there are relatively few studies on this.[26] The present study complements previous findings by bringing new information about stress generated in resin volumes for the BOT technique. These stress values can be useful for future studies and for understanding how this structure may fail clinically, in some cases compromising orthodontic treatment.
BOT has already been discussed in the literature with reports of more than 9 years of clinical success[3] and is considered a technique which is capable of ensuring good dental control. The present study supports this statement through the numerical data calculated during the computational analysis.
However, force control, adequate orthodontic planning, correct wire preparation, and composite resin modeling are essential, as are the occlusion type in each case and following a safe tooth movement protocol.[27]
FEA is a useful method for quantifying forces, moments and stresses, as well as other variables which enable orthodontic appliance activations to be simulated for dental movement in the three-dimensional coordinates.[28] Despite this wide use, the three-dimensional model used in this study is simplified, but it proved to be a useful tool to study and understand the stress distributions present similar to those in other published studies. This work is in agreement with a previous study which suggested that comparative computer simulations be used to evaluate complex cases with this methodology.[27-29]
As in most computer simulations of biological situations, the limitations of the study are mainly related to material parameters. It should be noted that the mechanical properties of biological tissues can vary from individual to individual and from region to region within the same organism.[13] As reported in the previous studies, the applied parameters do not perfectly reproduce the complex dental structure and behavior, bone tissue, and periodontal ligament. However, this method is useful to theoretically describe the initial stress distribution of maxillary incisor orthodontic intrusion and that the results could be considered in clinical treatment planning.[13,29] Despite the promising results reported in the present study, FEA has methodological limitations and therefore cannot replace clinical studies.[29,30] FEA is a controlled simulation and is widely used to calculate stress distributions under complex conditions.[16,30] Other advantages of this method over other research methodologies are the low laboratory costs, the reduced time to carry out the investigation and provides information which cannot be obtained by in vitro or even clinical studies involving this alternative technique.[30-34]
In addition, bonded orthodontic wire can produce reciprocal movement of the adjacent teeth, promoting consecutive changes in the transverse position and torque of teeth, and skewing of the arch form.[35] This should be considered a study’s limitation since the present study simulated just one tooth and variations in the wire shape will modify the biomechanical response. Another important limitation is that there is no validation report of the finite element method in the present study, which is essential for a safer clinical extrapolation. Finally, the models simulated herein have limitations such as isotropy, absence of occlusal forces, parafunction, and pH and temperature variation. Therefore, further studies should be carried out to deny of approve the current hypothesis.
CONCLUSION
Based on the results calculated in this study and considering the limitations present, we can conclude that:
Different magnitudes of stress concentration and deformation proportional to the orthodontic wire diameter were calculated in 1.0 mm buccal orthodontic movement in maxillary central incisors with BOT.
The induced biomechanical response in all simulated models was favorable for the applied displacement, regardless of the composite resin used.
Declaration of patient consent
Patient’s consent not required as there are no patients in this study.
Financial support and sponsorship
Nil.
Conflicts of interest
There are no conflicts of interest.
References
- Técnica ortodôntica fixa sem bráquetes, com tecnologia tridimensional “3D-BOT”. Sci Pract. 2019;12:22-30.
- [CrossRef] [Google Scholar]
- Anterior torque correction with bracketless fixed orthodontics. J Clin Orthod. 2012;46:558-62.
- [Google Scholar]
- The Bracket-less fixed orthodontics: Nine years of clinical experimentation. Prog Orthod. 2008;9:72-92.
- [Google Scholar]
- Lingual active retainers to achieve teeth levelling in orthodontics: Case series. Int Dent SA. 2008;10:24-9.
- [Google Scholar]
- 3D-BOT-a new alternative for aesthetic comfortable and predictable treatments-case reports. Orthod Sci Pract. 2019;12:47-59.
- [CrossRef] [Google Scholar]
- Revolution in orthodontics: Finite element analysis. J Int Soc Prev Community Dent. 2016;6:110-4.
- [CrossRef] [PubMed] [Google Scholar]
- Influence of preparation design, restorative material and load direction on the stress distribution of ceramic veneer in upper central incisor. Braz Dent Sci. 2021;24(3)
- [CrossRef] [Google Scholar]
- Simulation of mouthguard use in preventing dental injuries caused by different impacts in sports activities. Sport Sci Health. 2019;15:85-90.
- [CrossRef] [Google Scholar]
- Influence of different restorative material and cement on the stress distribution of ceramic veneer in upper central incisor. Indian J Dent Res. 2020;31:236-40.
- [CrossRef] [PubMed] [Google Scholar]
- Polymerization shrinkage stresses in a premolar restored with different composite resins and different incremental techniques. J Adhes Dent. 2013;15:341-50.
- [Google Scholar]
- Numerical simulation of the force generated by a superelastic NiTi orthodontic archwire during tooth alignment phase: Comparison between different constitutive models. Mater Res Express. 2018;5:45405.
- [CrossRef] [Google Scholar]
- Initial force systems during bodily tooth movement with plastic aligners and composite attachments: A three-dimensional finite element analysis. Angle Orthod. 2015;85:454-60.
- [CrossRef] [PubMed] [Google Scholar]
- Orthodontic intrusion of maxillary incisors: A 3D finite element method study In: Dental Press J Orthod. Vol 21. 2016. p. :75-82.
- [CrossRef] [PubMed] [Google Scholar]
- Scientific use of the finite element method in orthodontics In: Dental Press J Orthod. Vol 20. 2015. p. :119-25.
- [CrossRef] [PubMed] [Google Scholar]
- Wolff 's Law and bone's structural adaptations to mechanical usage: An overview for clinicians. Angle Orthod. 1994;64:175-88.
- [Google Scholar]
- Reduced periodontal support for lower central incisor-a 3D finite element analysis of compressive stress in the periodontium. J Int Acad Periodontol. 2021;23:65-71.
- [Google Scholar]
- Do mechanical advantages exist in relining fiber posts with composite prior to its cementation? J Adhes Dent. 2018;20:511-8.
- [Google Scholar]
- Effect of arch wire size on orthodontic reverse closing loop and retraction force in canine tooth distalization: Three-dimensional finite element analysis. J Orofac Orthop. 2019;80:17-24.
- [CrossRef] [PubMed] [Google Scholar]
- Short communication: Influence of restorative material and cement on the stress distribution of posterior resin-bonded fixed dental prostheses: 3D finite element analysis. J Mech Behav Biomed Mater. 2019;96:279-84.
- [CrossRef] [PubMed] [Google Scholar]
- Biological reaction of alveolar bone to orthodontic tooth movement. Angle Orthod. 1999;69:151-8.
- [Google Scholar]
- Comparison of orthodontic techniques and a suggestion of treatment for the athlete patient. Disciplinarum Sci Saúde. 2020;21:267-83.
- [CrossRef] [Google Scholar]
- Association of orthodontic force system and root resorption: A systematic review. Am J Orthod Dentofacial Orthop. 2015;147:610-26.
- [CrossRef] [PubMed] [Google Scholar]
- Finite element analysis of archwire parameters and activation forces on the M/F ratio of vertical, L-and T-loops. BMC Oral Health. 2020;20:70.
- [CrossRef] [PubMed] [Google Scholar]
- Current aspects on bonding effectiveness and stability in adhesive dentistry. Aust Dent J. 2011;56(Suppl 1):31-44.
- [CrossRef] [PubMed] [Google Scholar]
- The use of different adhesive filling material and mass combinations to restore Class II cavities under loading and shrinkage effects: A 3D-FEA. Comput Methods Biomech Biomed Eng. 2020;24:485-95.
- [CrossRef] [PubMed] [Google Scholar]
- Finite element study on modification of bracket base and its effects on bond strength In: Dental Press J Orthod. Vol 20. 2015. p. :76-82.
- [CrossRef] [PubMed] [Google Scholar]
- Interventions for managing relapse of the lower front teeth after orthodontic treatment. Cochrane Database Syst Rev. 2013;9:CD008734.
- [CrossRef] [PubMed] [Google Scholar]
- Comparison of two asymmetric headgear force systems: A finite element analysis In: Dental Press J Orthod. Vol 24. 2019. p. :41.e1-6.
- [CrossRef] [PubMed] [Google Scholar]
- Distribution of stress on TMJ disc induced by use of chincup therapy: Assessment by the finite element method In: Dental Press J Orthod. Vol 22. 2017. p. :83-9.
- [CrossRef] [PubMed] [Google Scholar]
- Comparative numerical analysis between two types of orthodontic wire for the lingual technique, using the finite element method. Appl Bionics Biomech. 2021;2021:6658039.
- [CrossRef] [PubMed] [Google Scholar]
- Treatment of Class II malocclusion with the 3dbot technique (Three-dimensional fixed orthodontics without brackets and skeletal anchorage) Orthod Sci Pr. 2020;13:29-35.
- [CrossRef] [Google Scholar]
- Influence of orthodontic movement by bracket-less orthodontic treatment on stress distribution: 3D finite element analysis. Curr Res Dent. 2021;12:48-61.
- [CrossRef] [Google Scholar]
- Evaluation of the effects of the dental and skeletal anchored face mask therapies on the craniofacial system by using nonlinear finite element analysis. APOS Trends Orthod. 2017;7:267-72.
- [CrossRef] [Google Scholar]
- Evaluation of stress on the periodontium induced by a fixed retraction screw appliance and an active tie-back: A 3-dimensional finite element study. APOS Trends Orthod. 2013;3:178-83.
- [CrossRef] [Google Scholar]
- Lingual bonded retainers: A case series of complications and resolutions. APOS Trends Orthod. 2020;10:3-11.
- [Google Scholar]