Translate this page into:
Evaluation and comparison of the impact of titanium dioxide nanocoating on frictional resistance of orthodontic stainless-steel wires: An experimental study
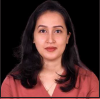
*Corresponding author: Karthika Edassery Suresh, Department of Orthodontics and Dentofacial Orthopedics, Sri Siddhartha Dental College, Tumakuru, Karnataka, India. edasserykarthika@gmail.com
-
Received: ,
Accepted: ,
How to cite this article: Suresh KE, Manjula KT, Kumar DC, Shailaja AM, Handique S. Evaluation and comparison of the impact of titanium dioxide nanocoating on frictional resistance of orthodontic stainless-steel wires: An experimental study. APOS Trends Orthod. doi: 10.25259/APOS_217_2024
Abstract
Objectives
The reduction in frictional force during sliding mechanics results in accelerated tooth movement and enhanced anchorage control. Recently, coating archwires with various nanoparticles has been suggested to lower frictional forces. In the present study, the primary objective is to evaluate and compare the efficiency of titanium dioxide (TiO2) nanocoated stainless-steel (SS) wires versus uncoated SS wires in reducing frictional resistance during sliding mechanics, also to assess and compare the surface roughness of TiO2 nanocoated SS wires and uncoated SS wires.
Material and Methods
Eighteen straight SS wires of 0.019 × 0.025 inches were divided into, experimental and control groups. The experimental group was coated with TiO2 nanoparticles and analyzed for surface roughness using an optical profilometer. Frictional resistance and coefficient of friction at 0.5 N and 1 N between the wires and brackets 0.022” slot size were tested using a texture analyzer.
Results
Uncoated SS wire exhibited significantly higher surface roughness (0.28 ± 0.06 Ra) compared to TiO2 coated wire (0.037 ± 0.09 Ra). The mean frictional resistance at 0.5 N and 1 N for coated wires were 2.15 ± 0.35 and 2.79 ± 0.30, respectively, while for uncoated wires, they were 1.15 ± 0.22 and 1.64 ± 0.84, indicating higher friction in coated wires. Surface analysis showed the coating peeling off after the friction test.
Conclusion
Increased frictional resistance between the wire and bracket slot in the experimental group can be contributed due to the peeling off of the coating, to strengthen the evidence on the impact of coated wires on frictional characteristics, more research is required.
Keywords
Titanium dioxide nanocoating
Frictional resistance
Radiofrequency sputtering
Surface roughness
Scanned electron microscopy
INTRODUCTION
Friction (FR) is defined as the resistive force between surfaces that opposes motion.[1] When two objects come in contact, the force works in the opposite direction of the moving body or surface, slowing or resisting their relative motion.[2] Friction is contributed by multifactorial characteristics that include both biological and mechanical variables. Sliding mechanics used for closing extraction space result in relative movement to occurs between the archwire and bracket interface resulting in frictional resistance.[3] Frictional resistance modifies the moment-to-force ratio of teeth and rotational center, hence influencing the orthodontic tooth movement rate and raising the risk of anchoring loss.[4]
Surface roughness is the trait of the material, shelf life, and manufacturing process. A smooth surface results in a vast area of adhesion that increases friction during sliding motion and a rough surface creates significant friction due to contact and interlocking between the peaks of the surface and the valley.[5] Various techniques are available to overcome friction during orthodontic tooth movement these include the use of extraoral force, temporary anchorage devices, or archwires of different materials, sizes, and shapes. Nanoparticles were used as a method to reduce the friction between metallic surfaces in the form of solid lubricant.[6]
One way to modify the material surface is through coating. Different coating techniques and materials have been used to enhance the surface properties of archwires. Nevertheless, some problems with coatings have been observed. Investigations are continuing to find appropriate materials and techniques with the objective of improving the properties of metallic biomaterials. Elhelbawy and Ellaithy showed that the coating of stainless steel (SS) orthodontic brackets and wires by nanoparticles of Chitosan or Zinc oxide (ZnO) during frictional test reduced the frictional force significantly resulting in better anchorage control, reduced treatment time, and risk of root resorption.[7] It was demonstrated by Ghasemi et al.[8] that titanium dioxide (TiO2)-coated brackets substantially reduced surface roughness which, in turn, reduced the colonization of Streptococcus mutans. Arici et al. suggests that the coefficients of friction of metal bracket–archwire combinations used in orthodontic treatment can be decreased by coating with Al2O3 and TiN thin film.[9] Orthodontic archwires are coated with nanoparticles to influence their surface features and, consequently, their properties. Such a coating can be done using TiO2. Radiofrequency (RF) magnetron sputtering is an effective technique on account of its inherent versatility, low-temperature deposition, and uniform surface coverage. The main advantage of this method is the ability to apply a thin, inorganic, and nanoparticle coating with an adjustable shape and size by tuning preparation parameters.[10] Therefore, the present study was designed to assess and compare the frictional resistance, and surface roughness of TiO2 nanoparticles coated SS straight wire with conventional SS wire, and the film integrity of the coating after undergoing a frictional test done with RF magnetron sputtering technique. The current study tested the null hypothesis pointing no difference between frictional resistance and surface roughness generated by TiO2 nanocoated stainless steel wire and conventional stainless steel wires.
MATERIAL AND METHODS
The study was conducted in Sree Chitra Tirunal Institute of Medical Science and Technology, Biomedical Technology wing, Trivandrum, Kerala.
Eighteen rectangular straight SS archwires of 0.019 × 0.025 inches were divided into two groups. The experimental group consisted of nine wires coated with TiO2 by RF magnetron sputtering method compared with nine uncoated control groups [Figure 1].
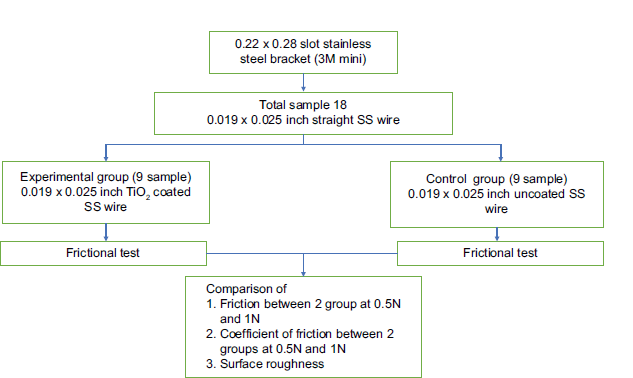
- Study design. SS: Stainless steel, TiO2: Titanium dioxide.
Samples size was calculated based on n = (Zα/2 + Zβ)2 × 2 × σ2/d2, Zα/2 = 1.96% at 95% confidence interval, Zβ = 2.33 at 99% power of the study, σ2 = 0.09, d = 0.6, n = 9.2 = 9 nos per group, with the final sample size of 9 + 9 = 18 nos to check the difference in frictional value and surface roughness.
Coating of the wire
RF magnetron sputtering was used to deposit a film of TiO2 nanocoating of thickness 800Ǻ on the experimental SS straight wire. The RF Sputtering system contains an SS deposition vacuum chamber. Substrates are placed in a vacuum chamber and are pumped down to a prescribed process pressure. Sputtering starts when a negative charge is applied to the target material causing a plasma or glow discharge. The negatively based target plate is quickly drawn to the positively charged gas ions produced in the plasma area. This collision creates a momentum transfer and ejects atomic-size particles from the target. As a result, these particles are applied as a thin layer on the surface of the substrate. After cleaning the experimental samples with acetone, the sample was placed in the base plate holder and secured with a ketone tape. Each sample was coated on one side and after coating on one side, the samples were flipped and kept for the same coating on the opposite side.
Frictional test
A single equivalent force acting at the root’s center of resistance was used to approximate the forces acting on the tooth root’s surface. The force acting on the archwire is counteracted by the couple created by the two-point contact.[11]
Eighteen specimens from both groups (length of 60 mm) were analyzed for the evaluation of frictional resistance at bracket and wire interface using Tidy’s protocol under dry conditions [Figure 2].[11] Four edgewise brackets (Victory Series, 3M Unitek) having a slot dimension of 0.022 × 0.028 inches were positioned at 8 mm intervals (inter bracket distance) on an acrylic plate for friction testing, with a 16 mm gap (retraction space) in the middle to allow the canine bracket to slide to replicate canine retraction.
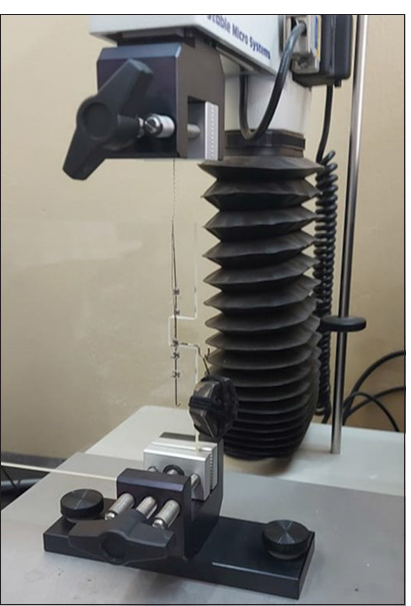
- Set up for evaluation of frictional test.
In the present study, rather than the bracket itself, a power arm was used to exert the force necessary for retraction. Power arm mechanics are used in the clinical setting, and they are replicated here. The experimental configuration of tidy serves as the foundation. However, due to the effects of binding and notching, the frictional resistance may vary when the force is applied directly to the bracket. Therefore, the findings can be applied in a clinical setting where retraction is accomplished using a power arm or an archwire hook.[12]
Initially, the ligature on the movable bracket was tightened completely and then loosened to allow free sliding. The movable bracket was equipped with a 12 mm power arm, from which weights of 0.5 N and 1 N were hung to simulate the single equivalent force acting at the tooth root’s center of resistance. The power arm’s length was selected to represent the distance from the slot to the center of resistance of a typical canine tooth. Metallic ligatures measuring 0.010 inches were used to secure the wires.
All tests were conducted under dry conditions, texture analyzer (Model and manufacturer: TA.XT Plus [Stable Micro Systems, UK]) was used for the study with a movable bracket suspended from the load cell of the testing machine, while the base plate (Perspex sheet) was mounted on the crosshead below.
Tooth movement typically progresses at a rate of around 1 mm per month, corresponding to an average speed of approximately 2.3 × 10–5 mm per minute. For the experiment, a speed of 5 mm/min was selected, as higher speeds did not accurately reflect real clinical conditions. A study examining the effect of varying crosshead speeds (ranging from 0.5 to 50 mm/min) on frictional resistance using an Instron universal testing machine found no significant differences in the results.[13]
The load cell reading represents the clinical force of retraction that would be applied to the canine, part of which would be critical friction while the rest would be the translation force on the tooth. The difference between the load cell reading and the load on the power arm represents frictional resistance. The coefficient of friction at the wire-bracket interface was calculated using the appropriate formula, P = 2Fhm/W, where (P: Frictional resistance, F: Equivalent force acting at a distance, W: Bracket slot width, h: 12 mm, and m: Coefficient of friction).
Scanning electron microscopy (SEM)
SEM attached with an energy-dispersive X-ray analysis system with OXFORD Link Ultracool Detector, having a resolution of 133eV/Mn was used to check the surface of the coated wire and the uniformity of the coating. Samples were mounted using a suitable grip and analysis was performed at different magnifications ×100, ×500, and for both coated and uncoated wire [Figure 3a and b].
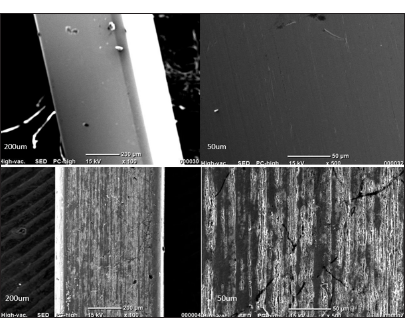
- (a) Scanned electron microscopy of uncoated stainless steel wire at magnification of ×100 and ×500. (b) Scanned electron microscopy of titanium dioxide nanocoated stainless steel wire at magnification of ×100 and ×500.
Surface roughness test
The TiO2 coated and uncoated wires were subjected to a surface roughness test using optical profiling (VEECO TECHNOLOGIES VS1000). The samples were mounted on the wedge using a suitable grip and a test was conducted. After the samples were mounted, optical profiling analysis was performed using the Vecco Surface profiler. Crosshead is selected with speed for scan length 1 um × 1 um. To determine the average roughness using a surface profilometer, 1.6 mm × 1.6 mm samples were obtained from the area where canine bracket sliding occurred. Samples were set with the American Society for Testing Materials standard: ISO4287. Surface roughness data reported in Arithmetic Roughness Ra Root Mean Square Rq in um scale.
TiO2 film integrity
SEM before and after the frictional test at ×100, ×300, and ×1000 was done to the test material obtained from the part of the wire that underwent canine retraction, to check the integrity of TiO2 nanocoating after the frictional test [Figure 4].
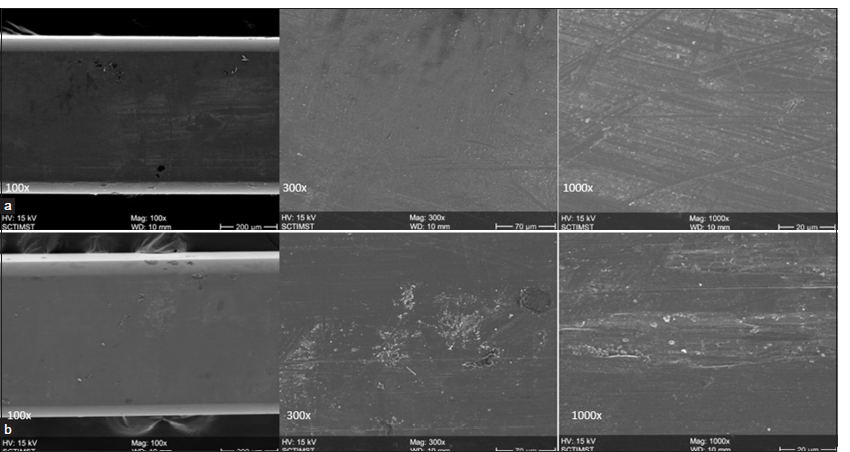
- (a) Scanning electron microscopy (SEM) picture of test material obtained from part of the wire that underwent canine retraction before frictional test at ×100, ×300, and ×1000. (b) SEM picture of test material obtained from part of the wire that underwent canine retraction after frictional test at ×100, ×300, and ×1000.
RESULTS
The present study compared the frictional value of coated TiO2 and uncoated SS wires. The data were collected, coded, and fed in the Statistical Package for the Social Sciences (IBM version 23) for statistical analysis. The descriptive statistics included mean and standard deviation. The inferential statistics included an Independent “t”-test for comparison between the two groups. The level of significance was set at 0.05 at a 95% confidence interval.
Frictional resistance
In the present study, evaluation of frictional resistance at bracket and wire interface was conducted under dry conditions using TiO2 nanocoated wire at 0.5 N and 1 N weight showed frictional resistance and coefficient of friction of 2.14 ± 0.35 N and 0.18 ± 0.05 with 0.5N [Table 1] and frictional resistance of 2.79 ± 0.30N and coefficient of friction of 0.16 ± 0.09 with 1 N [Table 2]. The uncoated SS wire showed a frictional resistance of 1.15 ± 0.22 N and coefficient of friction of 0.16 ± 0.01 with 0.5 N of weight [Table 3] and a frictional resistance of 1.63 ± 0.84N and a coefficient of friction of 0.14 ± 0.03 with 1 N of weight [Table 4]. The results indicated higher values of frictional resistance due to the proportional relationship between friction and applied load, while the coefficient of friction remained relatively constant for given material pairs. This suggests that as the load increases, the frictional resistance also increases, but the inherent material properties (coefficient of friction) do not change significantly. Therefore, the frictional resistance can be high even if the coefficient of friction is low, depending on the applied load.
Mean | Standard deviation | t-value | Sig. | ||
---|---|---|---|---|---|
Frictional resistance | |||||
0.5 N | 2.14 | 0.35 | −4.16 | 0.001 (HS) | |
Coefficient of friction | |||||
0.5 N | 0.18 | 0.005 | 5.84 | 0.000 (HS) |
Sig.: Significance, HS: Highly statistically significant
Mean | Standard deviation | t-value | Sig. | |
---|---|---|---|---|
Frictional resistance | ||||
1 N | 2.79 | 0.30 | −4.16 | 0.001 (HS) |
Coefficient of friction | ||||
1 N | 0.16 | 0.008 | 5.84 | 0.000 (HS) |
Sig.: Significance, HS: Highly statistically significant
Mean | Standard deviation | t-value | Sig. | |
---|---|---|---|---|
Frictional resistance | ||||
0.5 N | 1.15 | 0.22 | −1.64 | 0.120 (NS) |
Coefficient of friction | ||||
0.5 N | 0.16 | 0.01 | 1.34 | 0.197 (NS) |
Sig.: Significance, HS: Highly statistically significant
Mean | Standard deviation | t-value | Sig. | |
---|---|---|---|---|
Frictional resistance | ||||
1 N | 1.63 | 0.84 | −1.64 | 0.120 (NS) |
Coefficient of friction | ||||
1 N | 0.14 | 0.03 | 1.34 | 0.197 (NS) |
Sig.: Significance, HS: Highly statistically significant
Frictional resistance generally increased with greater applied normal force for both dry and wet conditions, but the mean coefficients of friction are higher in wet conditions due to increased atomic attraction among ionic species. Stannard et al. stated that the average coefficient of variation is 12.56% for dry conditions and 14.88% for wet conditions, indicating more dispersion in the wet conditions.[14]
On comparison of the mean frictional resistance at 0.5 N, a statistically significant difference between TiO2 coated SS wire and uncoated SS wire (P < 0.05, P = 0.000) was seen. With 1 N comparing the mean frictional resistance showed a highly significant difference between TiO2 coated SS wire and uncoated SS wire (P < 0.05, P = 0.001) [Table 5 and Graph 1].
Mean | Standard deviation | t-value | Sig. | |
---|---|---|---|---|
0.5 N | ||||
TiO2 SS coated wires | 2.146778 | 0.3535738 | 7/046 | 0.000 (HS) |
Uncoated SS coated wires | 1.158667 | 0.2280433 | ||
1 N | ||||
TiO2 SS coated wires | 2.796000 | 0.3069446 | 3.847 | 0.001 (HS) |
Uncoated SS coated wires | 1.639000 | 0.8485027 |
TiO2: Titanium dioxide, SS: Stainless-steel, Sig.: Significance, HS: Highly statistically significant
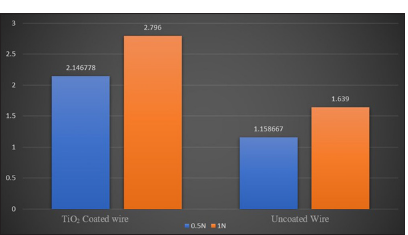
- Mean frictional resistance at 0.5 N and 1 N in titanium dioxide stainless steel coated wire and uncoated. X-axis represent titanium dioxide coated stainless steel wire and uncoated stainless steel wire at 0.5 N and 1N. Y-axis shows the mean frictional resistance.
Surface roughness test
The physical appearance of the surface shows a uniform dense titanium coating layer under SEM and relatively smooth morphology for coated samples when compared to uncoated samples, indicating no abrasion/pitting, which was in accordance with the findings of Anuradha et al. [15]
When a bandpass of the specimen is selected using an optical profilometer, a filtered surface is obtained showing the surface roughness. This texture diffuses light from different angles. Proper spatial filtering reveals through mean roughness differ between mirrors. An increase in amplitude roughness also increases the diffusion of light and this diffusion directly scopes with efficiency of reflectivity. These show mean roughness parameters to quantify the appearance and reflectivity efficiency of the mirrors [Figures 5 and 6].[16]
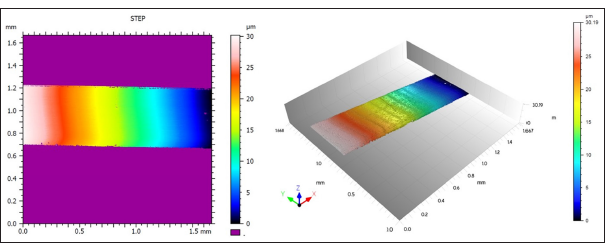
- Optical profilometer test showing uncoated stainless steel wire.
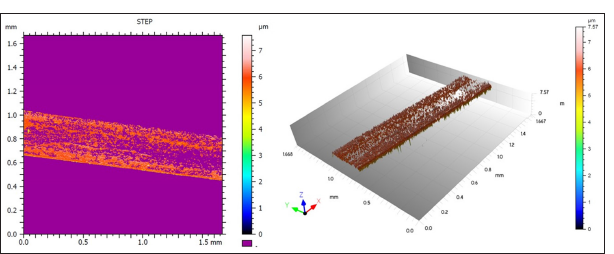
- Optical profilometer test showing titanium dioxide nanocoated stainless steel wire.
The mean surface roughness of TiO2 nanocoated wires and uncoated SS wire was 0.037 ± 0.09 Ra and 0.28 ± 0.06 Ra, respectively. There was a statistically significant difference noted when comparing between two types of wires (P < 0.05, P = 0.000). The surface roughness of TiO2 nanocoated SS wire is better than uncoated SS wire [Table 6 and Graph 2 ].
Mean | Standard deviation | t-value | Sig. | |
---|---|---|---|---|
TiO2 nano coated wires | 0.037 | 0.008 | -11.315 | 0.000 (HS) |
Uncoated SS wires | 0.281 | 0.06 |
TiO2: Titanium dioxide, SS: Stainless-steel, Sig.: Significance, HS: Highly statistically significant
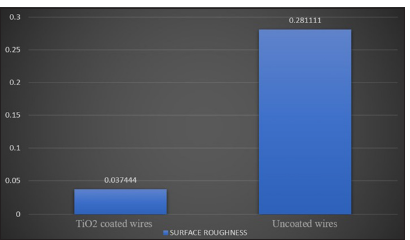
- Mean surface roughness of titanium dioxide stainless steel coated wire and uncoated wire. X-axis represents Titanium dioxide coated and Uncoated wires and Y-axis represent the mean surface roughness.
TiO2 film integrity
Figure 3 shows a scanning electron micrograph of uncoated and TiO2 coated wire at ×100, ×500 magnification. The coated wires showed the presence of titanium peaks only, indicating the absence of any secondary phase formation during the sputtering process.
SEM before and after the frictional test at ×100, ×300, and ×1000 showed delamination, deterioration, and peeling off of the coating in the TiO2 nanocoated group [Figure 4].
DISCUSSION
The frictional force between the bracket and the wire is a crucial aspect of clinical orthodontics. Reducing this frictional force can enhance the efficiency of tooth movement, thereby shortening the treatment duration.
Nevertheless, during the sliding process, friction between the bracket and wire interface is unavoidable, resulting in the application of greater forces to overcome the friction, which can result in anchorage loss.[17] Previous studies have shown a reduction in surface roughness and friction on the coating of archwires with a diamond-like carbon coating, ZnO, Zirconium oxide, Teflon, and inorganic fullerene-like tungsten nanoparticles.[16-28] Hence, the present study was conducted to evaluate the surface roughness and efficiency of TiO2 nanocoated SS wires for reduction of frictional resistance during sliding mechanics and the persistence of coating after the frictional test using RF magnetron sputtering technique.
Furthermore, most studies on nanoparticle-coated TiO2 have focused on bacterial adhesion using the photocatalytic activity of TiO2. In the present study, a surface roughness test was carried out using optical profiling (VEECO TECHNOLOGIES VS1000) for TiO2 coated SS wires and to compare with uncoated SS wires. Our study results showed increased surface roughness of 0.28 ± 0.06 uncoated SS wire when compared with TiO2 nanocoated SS wire 0.03 ± 0.08, which was in accordance with the study by Venkatesan et al. indicating that coated wires were smoother compared to uncoated wires.[28] A similar study done by Anuradha et al. reported that sputter coating with TiO2 particles on the archwires decreased the surface roughness.[15,28] A systemic review by Solanki et al. concluded that TiO2 coated brackets showed reduced surface roughness, minimal bacterial adhesion, and less cytotoxic activity.[26]
Ghasemi et al. utilized titanium oxide and silver oxide nanofilms of different thicknesses (60 and 100 um) and found that friction among silver oxide-coated brackets was lower than that of the titanium oxide and uncoated brackets; also, atomic force microscopy showed smoother surface in coated brackets.[8] The friction on brackets with titanium oxide coating increased at both 60 and 100 um thickness. The present results are incongruent with the above. On evaluation of the effect of RF magnetron sputtered TiO2 wires on frictional resistance, results showed an increase in frictional resistance and coefficient of friction in both 0.5 N and 1 N groups. The mean frictional resistance at 0.5N of TiO2 coated SS wire and uncoated SS wire was 2.15 ± 0.35 and 1.15 ± 0.22, respectively. The mean frictional resistance at 1 N of TiO2 coated SS wire and uncoated SS wire was 2.79 ± 0.30 and 1.64 ± 0.84, respectively. In addition, the SEM image after the frictional test showed peeling off of the coating. This finding was in accordance with Venkatesan et al. and Arici et al.[9,28] On the evaluation of frictional resistance and peeling of coating among titanium coated arch wires, Chaturvedi et al., showed reduced friction among SS wire coated with sol gel concentration of 1:2–1:6 for a duration of 48 h.[29] In contrast, Ghasemi et al. coated SS wire with titanium oxide using a physical vapor deposition technique which showed increased friction with both 60 um and 100 um thickness; similarly, Arici et al. used TiN to coat SS wire with RF/Direct current (DC) magnetron sputtering and found peeling off the coating after the frictional test.[8,9] In the present study, the increased frictional resistance between the wire and bracket interface can be contributed due to the peeling off of the coating, the residual coating material at the point of contact point between the surfaces could be the reason for the increased friction. This peeling can be contributed to thermal expansion differences between the base material and coating materials similar to the study done by Arici et al.[9] In a recent study, the surface of chromium-nickel (Cr-Ni) archwires was examined in relation to silicon dioxide and TiO2 nanoparticles. The results indicated that TiO2 did not significantly reduce friction and TiO2 coating promoted the most heterogeneous surface morphology of Cr-Ni orthodontic wire.[30] Previous studies show that the coating technique and conditions play an important role in determining the frictional characteristics of the end product.[29]
For the reduction of frictional resistance, the present study used the RF magnetron sputtering technique to coat TiO2 on SS wire, however following the frictional test, the coating peeled off resulting in an increase in frictional resistance. Study results revealed higher friction when compared to the sol gel technique, a study by Chaturvedi et al. indicating that the sol gel method is a better choice when compared with the RF sputtering method in coating TiO2.[29] In a systemic review by Zhang et al., the deformation and peeling of the surface morphology was one of the reasons for greater frictional force among TiO2 coated brackets.[31] Similarly, the application of TiO2 coatings on SS brackets using PVD did not reduce friction.[32]
Limitation of the study
Further research is to be done by altering the size of nanoparticles and the thickness of the coating.
CONCLUSION
TiO2 nanocoated SS wires showed a reduction in surface roughness compared to uncoated SS wire under an optical profilometer. The frictional test among the groups showed increased frictional resistance with TiO2 nanocoated wire compared to uncoated SS wire done with the RF sputtering technique.
Ethical approval
The research/study was approved by the Institutional Review Board at Sri Siddhartha Medical College, number SSMC/Dent/IEC-44/May 2022, dated May 17, 2022.
Declaration of patient consent
Patient consent was not required as there are no patients in this study.
Conflicts of interest
There are no conflicts of interest.
Use of artificial intelligence (AI)-assisted technology for manuscript preparation
The authors confirm that there was no use of artificial intelligence (AI)-assisted technology for assisting in the writing or editing of the manuscript and no images were manipulated using AI.
Financial support and sponsorship: Nil.
References
- Friction and resistance to sliding in orthodontics: A critical review. Am J Orthod Dentofacial Orthop. 2009;135:442-7.
- [CrossRef] [PubMed] [Google Scholar]
- Frictional forces between bracket and arch wire. Am J Orthod Dentofacial Orthop. 1989;96:397-404.
- [CrossRef] [PubMed] [Google Scholar]
- Friction in perspective. Am J Orthod Dentofacial Orthop. 1999;115:619-27.
- [CrossRef] [PubMed] [Google Scholar]
- Frictional resistance between orthodontic brackets and archwires in the buccal segments. Angle Orthod. 1996;66:215-22.
- [Google Scholar]
- Static frictional force and surface roughness of nickel-titanium arch wires. Am J Orthod Dentofacial Orthop. 1991;100:341-8.
- [CrossRef] [PubMed] [Google Scholar]
- Debris, roughness and friction of stainless steel archwires following clinical use. Angle Orthod. 2010;80:521-7.
- [CrossRef] [PubMed] [Google Scholar]
- Comparative evaluation of Stainless-steel wires and brackets coated with nanoparticles of Chitosan or Zinc oxide upon friction: An in vitro study. Int Orthod. 2021;19:274-80.
- [CrossRef] [PubMed] [Google Scholar]
- Antimicrobial effect, frictional resistance, and surface roughness of stainless steel orthodontic brackets coated with nanofilms of silver and titanium oxide: A preliminary study. Microsc Res Tech. 2017;80:599-607.
- [CrossRef] [PubMed] [Google Scholar]
- Effectiveness of medical coating materials in decreasing friction between orthodontic brackets and archwires. Korean J Orthod. 2021;51:270-81.
- [CrossRef] [PubMed] [Google Scholar]
- Functional coatings for orthodontic archwires-a review. Materials (Basel). 2020;13:3257.
- [CrossRef] [PubMed] [Google Scholar]
- Frictional forces in fixed appliances. Am J Orthod Dentofacial Orthop. 1989;96:249-54.
- [CrossRef] [PubMed] [Google Scholar]
- Comparison of frictional resistance between four types of brackets in combination with stainless steel and beta-titanium archwires. APOS Trends Orthod. 2020;10:231-7.
- [CrossRef] [Google Scholar]
- Effect of bracket and wire composition on frictional forces. Eur J Orthod. 1991;13:322-8.
- [CrossRef] [PubMed] [Google Scholar]
- Comparative friction of orthodontic wires under dry and wet conditions. Am J Orthod. 1986;89:485-91.
- [CrossRef] [PubMed] [Google Scholar]
- Reliability performance of titanium sputter coated Ni-Ti arch wires: Mechanical performance and nickel release evaluation. Biomed Mater Eng. 2015;26:67-77.
- [CrossRef] [PubMed] [Google Scholar]
- Surface roughness measurement In: Speckle metrology. New York: Academic Press; 1978. p. :11-49.
- [CrossRef] [Google Scholar]
- A comparative study of frictional forces between orthodontic brackets and arch wires. Am J Orthod Dentofacial Orthop. 1991;100:513-22.
- [CrossRef] [PubMed] [Google Scholar]
- In vitro evaluation of physical vapor deposition coated beta titanium orthodontic archwires. Angle Orthod. 2012;82:22-9.
- [CrossRef] [PubMed] [Google Scholar]
- Improved orthodontic stainless steel wires coated with inorganic fullerene-like nanoparticles of WS(2) impregnated in electroless nickel-phosphorous film. Dent Mater. 2008;24:1640-6.
- [CrossRef] [PubMed] [Google Scholar]
- Effects of a diamond-like carbon coating on the frictional properties of orthodontic wires. Angle Orthod. 2011;81:141-48.
- [CrossRef] [PubMed] [Google Scholar]
- Effect of zirconium oxide nano-coating on frictional resistance of orthodontic wires. J Orthod Sci. 2022;11:35.
- [CrossRef] [PubMed] [Google Scholar]
- The effect of Teflon coating on the resistance to sliding of orthodontic archwires. Eur J Orthod. 2012;34:410-7.
- [CrossRef] [PubMed] [Google Scholar]
- The effect of zinc oxide nanoparticles deposition for friction reduction on orthodontic wires. Dent Res J (Isfahan). 2013;10:499-505.
- [Google Scholar]
- The effect of ZnO nanoparticle coating on the frictional resistance between orthodontic wires and ceramic brackets. J Dent Res Dent Clin Dent Prospects. 2016;10:106-11.
- [CrossRef] [PubMed] [Google Scholar]
- Zinc-oxide nanocoating for improvement of the antibacterial and frictional behavior of nickel-titanium alloy. Nanomedicine (Lond). 2016;11:2511-27.
- [CrossRef] [PubMed] [Google Scholar]
- Effects of nanostructured, diamondlike, carbon coating and nitrocarburizing on the frictional properties and biocompatibility of orthodontic stainless steel wires. Angle Orthod. 2016;86:782-8.
- [CrossRef] [PubMed] [Google Scholar]
- Effects of titanium oxide coating on the antimicrobial properties, surface characteristics, and cytotoxicity of orthodontic brackets - A systematic review and meta-analysis of in-vitro studies. J Oral Biol Craniofac Res. 2023;13:553-62. Erratum in: J Oral Biol Craniofac Res 2024; 14:360-1
- [CrossRef] [PubMed] [Google Scholar]
- Evaluation of titanium dioxide coating on surface roughness of nickel-titanium archwires and its influence on Streptococcus mutans adhesion and enamel mineralization: A prospective clinical study. Am J Orthod Dentofacial Orthop. 2020;158:199-208.
- [CrossRef] [PubMed] [Google Scholar]
- Evaluation of surface-modified orthodontic wires by different concentration and dipping duration of titanium oxide (TiO(2)) nanoparticles. J Orthod Sci. 2023;12:3.
- [CrossRef] [PubMed] [Google Scholar]
- Assessment of frictional resistance and surface roughness in orthodontic wires coated with two different nanoparticles. Microsc Res Tech. 2022;85:1884-90.
- [CrossRef] [PubMed] [Google Scholar]
- Functional surface coatings on orthodontic appliances: Reviews of friction reduction, antibacterial properties, and corrosion resistance. Int J Mol Sci. 2023;24:6919.
- [CrossRef] [PubMed] [Google Scholar]
- A novel method of coating orthodontic archwires with nanoparticles. J Int Oral Health. 2015;7:30-3.
- [Google Scholar]