Translate this page into:
The antimicrobial effect of doxycycline and doped ZnO in TiO2 nanotubes synthesized on the surface of orthodontic mini-implants
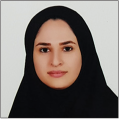
-
Received: ,
Accepted: ,
How to cite this article: Noorollahian S, Kachuie M, Hatamzade Z, Moghadam M, Narimani T. The antimicrobial effect of doxycycline and doped ZnO in TiO2 nanotubes synthesized on the surface of orthodontic mini-implants. APOS Trends Orthod 2022;12:162-7.
Abstract
Objectives:
Anchorage preservation is crucial in orthodontic treatment success. Mini-implants make a revolution in this domain. The failure of orthodontic mini-implants due to inflammation and infection is one of the reasons for anchorage loss. The purpose of this study was to evaluate the effect of a novel mini-implant surface modification to improve resistance against microbial contamination and surrounding tissue inflammation.
Material and Methods:
Twenty-four orthodontic mini-implants (Jeil Medical Corporation, Korea) with 1.6 mm diameter and 8 mm length were randomly divided into three groups: Group 1: Control group, Group 2: Nanotubes were made on the surface with anodisation, and Group 3: Zinc Oxide (ZnO) doped into nanotubes, and then doxycycline is added to them. The anti-bacterial efficacy against Porphyromonas gingivalis was evaluated using the disk diffusion method. To analyze data, Kruskal–Wallis, Friedman, and Wilcoxon tests were done. The significance level was set at 0.05.
Results:
No zone of the inhibition was formed in Groups 1 and 2. In Group 3, the mean (SD) diameter of the inhibition zone in the first 5-day to sixth 5-day were 38.7(8.2), 25(4.8), 17.8(5.6), 7.63(5.37), 1.5(2.83), and 0 millimeters, respectively.
Conclusion:
Nanotubes containing doped ZnO and Doxycycline are capable of preventing bacterial growth around the mini implant surfaces for at least up to 30 days. To manage inflammation of surrounding tissues of mini-implants, nanotubes are not effective alone. Therefore, the presence of diffusible materials in addition to nanotubes on the surface of mini-implants is necessary.
Keywords
Orthodontic mini-implant
Nanotube
Titanium
Doxycycline
Zinc oxide
INTRODUCTION
Anchorage preservation is one of the most challenging orthodontic issues defined as resistance to unwanted tooth movement.[1] In routine intra-oral methods, anchorage loss is always possible and is a great concern and extra-oral methods have limitations due to patient cooperation.[2]
The introduction of mini-implants as skeletal anchorage has allowed clinicians to overcome anchorage instability or dependence on patient cooperation.[2-4] Their advantages are the ease of insertion and removal, reasonable cost, biocompatibility, tolerating of most orthodontic forces, and acceptable success rate.[5,6] They facilitate complex tooth movements, for example, posterior teeth movements (intrusion, distalization, and protraction), intrusion of incisors, and cross-bite correction.[7-9]
The concern in the application of mini-implants is screw loosening and failure of it causes anchorage loss.[10] Factors related to the survival of mini-implants include host tissue-related, mini-implant-related, insertion procedure-related, loading-related factors, and patient oral hygiene.[11] Microbial infection of surrounding soft and hard tissues and following inflammation make bone loss and screw loosening. The role of bacteria as a prominent factor has been confirmed in peri-implantitis.[12] Soft-tissue integrity around dental implant protects alveolar bone from the oral environment. This barrier can be impaired by microbial penetration and plaque formation on the implant surface.[13] The following inflammation makes bone resorption and loosening of the implant, so maintaining hygiene around implants and preventing microbial colonization in this area is essential.[13,14]
Implant surface modification is an effective method to reduce implant-related infections.[15] Various techniques such as sandblasting, laser therapy, acid etching, hydroxyapatite coating, and anodization have been tested.[16-22] Anodizing creates the characteristics of regular nano-topography, like nano-tubes. It improves the biomechanical stability of in vivo porous titanium implants compared to other types of surface modification techniques.[23] Increasing surface [Figure 1] and lower contact angles with water create a hydrophilic surface that supports protein adhesion and improves the initial stage of osteointegration.[24-27] Studies showed that Titanium Oxide (TiO2) nano-tubes have antibacterial properties against Streptococcus mutans, Escherichia coli, and Staphylococcus.[15,28] Karmarker et al. showed anodizing improved removal torque of orthodontic mini-implants.[10]

- Increase surface nanotubes.
High concentrations of zinc show antibacterial properties.[29] The antibacterial mechanism of ZnO is mainly the production of reactive oxygen species that, due to their high reactivity, can destroy the integrity of the cell wall and cause bacterial death.[30] Petrini et al. showed that titanium surface chemically modified with ZnO significantly reduced five streptococcus species.[31] Pharmaceutical agents such as antibiotics add antibacterial properties to implant surfaces.[13] Doxycycline improves bone growth and the treatment of periodontal disease as well as peri-implantitis.[32] Considering that, the failure of mini-implants due to infection and inflammation caused by it, and considering that orthodontic treatments themselves cause significant changes in the bacterial environment of the mouth, which is associated with more gingivitis.[33,34]
This study aimed to investigate the surface modification and antimicrobial effect on orthodontic mini-implants.
MATERIAL AND METHODS
Twenty-four orthodontic mini-implants (Jeil Medical Corporation, Korea) with 1.6 mm diameter and 8 mm length were randomly divided into three groups: Group 1: Control group, Group 2: nanotubes were made on the surface with anodization, and Group 3: ZnO doped into nanotubes and then Doxycycline is added to them. The anti-bacterial efficacy against Porphyromonas gingivalis was evaluated using the disk diffusion method.
Preparing the surface of samples
Nanotube fabrication
Mini-implants in the second and third groups were cleaned by the ultrasound bath with an acetone alcohol solution (2-propanol) and washed with de-ionized water. An anodic oxidation process was performed in a magnetic stirrer glass reactor, in which a mini-implant as anode and platinum electrode was used as cathode. Only the head and half of the screw are immersed in the solution. Two electrodes with a distance of 3 cm were placed in an electrolyte solution composed of ammonium fluoride (NH4F). The process was continued at 60 v for 6 h at room temperature. The samples were washed using deionized water after the anodization process and dried with nitrogen gas. Then, the anodized samples were annealed at 450°C for 3 h. This changed the shapeless structure of TiO2 to a crystalline structure.[35] The surface morphology of samples was evaluated by scanning electron microscopy and see nanotubes of TiO2 have been created on it [Figure 2].

- SEM view of titanium oxide nanotubes (×20,000).
Incorporation of ZnO nanoparticles into Ti nanotube
In the third group, mini-implants were anodized, and then the ZnO was doped into nano-tubes to reach the concentration of 0.0150 M, then they were immersed in an aqueous solution containing nitrate on (Zn (NO3)2) and hexamethylenetetramine (CH2)6N4 with a molar ratio of 1:2.2 mg of citric acid was added to the solution. The samples passed hydrothermal reaction for 2 h at 70°C to form all the ZnO-decorated nano-tubes.[36]
Drug loading
In the following, for the third group, 100 mg (doxycyclineHyclate D9891, Sigma-Aldrich) to a solution of 10 wt% bovine gelatin (type B powder reagent, CAS 9000-70-8; Sigma-Aldrich) in de-ionized water and stirred for 1 h at 40°C, to obtain a homogenous drug/gelatin solution. Then each mini-implant was sonicated in gelatin/drug solution for 30 min to penetrate the drug/gelatin into nano-tubes. Each loaded mini-implant was left to dry at room temperature for 24 h.[29]
All samples were exposed to (UV) radiation for sterilization under a UV lamp with a power of 20 w and a distance of 10 cm from the lamp for 1 h.[28]
Culture of bacteria and antibiogram test
Antibacterial activity of the samples against the strain of P. gingivalis (ATCC 33277) was performed using the disk diffusion method.[14] Bacteria were cultured in tubes containing 5 ml of growth control medium under anaerobic conditions at 37°C for 48 h to reach bacteria in the mid-logarithmic phase. Then, bacterial suspensions were diluted to achieve the final density of 1.5 × 106 colonies per ml. Diluted suspension of bacteria (1 ml) was placed on the agar medium and allowed to dry for 10 min. Then, mini-implants of all three groups were placed on agar blood plates contaminated with bacteria. Plates containing culture medium were incubated under anaerobic conditions at 37°C. Then, the maximum diameter of the inhibitory zone was measured in mm after 5 days.[30] To evaluate the persistence of antibacterial properties, the samples in which the inhibitory zone was formed were transferred to the new plate every 5 days until no forming of the inhibitory zone was.
RESULTS
In the first and the second group, the inhibitory zone was not formed. In the third group, the mean and standard deviation of the diameter of inhibition zones is shown in [Table 1 and Figure 3]. Kruskal–Wallis test showed that there was a significant difference between the three groups based on different times (the first 5-day to the sixth 5-day). The calculated P-value in 5-day period intervals was: First 5-days to fourth 5-day 0.001 >PV, fifth 5-day 0.124 > PV, and sixth 5-days 0.1> PV.
5-Day Periods | ||||||
---|---|---|---|---|---|---|
1st | 2nd | 3rd | 4th | 5th | 6th | |
Min. | 30 | 20 | 10 | 0 | 0 | 0 |
Max. | 48 | 30 | 26 | 15 | 7 | 0 |
Mean | 38.7 | 25 | 17.8 | 7.63 | 1.5 | 0 |
Standard deviation | 2.83 | 4.8 | 5.6 | 5.37 | 2.83 | 0 |
P-value | 0 | 0 | 0 | 0/001 | 0/124 | 1 |

- (a) First 5-day: Sample from Group 3 (upper side), a sample from Group 2 (lower left), and sample from Group 1 (lower right). Samples of Group 3 in the following 5-days: (b) Second 5-day, (c) Third 5-day, (d) Fourth 5-day, (e) Fifth 5-day, and (f) sixth 5 days.
Friedman test showed that there was a significant difference between the first 5-days and the sixth 5-day (0.001 > PV) [Table 2]. Wilcoxon test showed that there was a significant difference between all-time intervals (P < 0.05) except for the fifth 5-day and sixth 5-day (P = 018).
DISCUSSION
Orthodontic mini-implants can provide reinforce anchorage, improve the success rate of treatments, and facilitate complex orthodontic treatments. Their effect on improving the quality of orthodontic treatments depends on their survival. Inflammation of the surrounding tissues of mini-implant is the most important factor in adjacent bone loss, reduction of mechanical retention, and ultimately failure of it. Therefore, any factor that prevents, reduces, or eliminates this inflammation improves the clinical success of mini-implants.[31,33]
The primary approach is preserving good oral hygiene of extra-tissue parts of mini-implants. In in vivo and in vitro studies, mechanical removal of bio-films around dental implants by air powder abrasion and titanium brush has not entirely removed the bio-films and about 10% of them have remained.[34] Therefore, mechanical methods of plaque control around mini implants are not enough to eliminate all bacterial bio-films. Another way is the use of local antimicrobial materials on the tissues around the mini-implant. This depends on the patient’s cooperation and is limited only to extra-tissue parts of mini-implants and intra-tissue parts are not in access.[14] Chen et al. showed the local application of hydro-gel containing ibuprofen and fibroblast growth factor can control inflammation and reduce the risk of peri-implantitis.[31]
Adding anti-inflammatory and antimicrobial agents to the mini-implant surface is the only way to be effective on both intra- and extra-tissue parts.[15] Creating nano-tubes on the surface of titanium mini-implants by the anodizing method have some advantages, for example, increasing surface area, the lower contact angle with water, antimicrobial properties on the mini-implant surface, improved protein adhesion, osteoblast reaction, and the initial stage of osteointegration.[10,24,27] Giordano et al. showed that surface anodization reduces the colonization of bacteria such as Staphylococcus aureus, Staphylococcus epidermidis, S. mutans, and P. gingivalis on the surface of implants.[37] Cui et al. stated that TiO2/bio-composite layers have excellent antibacterial activity against S. mutans.[21] Ercan et al. showed that titanium nano-tubes significantly reduced the formation of S. aureus biofilm on the surface after 2 days.[34] Titanium nanotubes have a high surface/volume ratio and negative electrical charge on their walls that make antibacterial properties and the potential to bind with positive proteins surface on osteoblasts.[35] Karmarker et al. showed that the maximum removal torque of mini-implants was higher in the anodized group, in an animal study, which promises better anchorage in orthodontic treatments.[10]
Nano-tubes reduce the colonization of bacteria on the surface of the implant and facilitate primary osteointegration. This reduces infection potential over time.[34] This means nano-tubes act as anti-microbial agents on the surface. However, in the present study, in the control group and mini-implants with nano-tubes, an inhibitory zone has not formed that indicates just the presence of nano-tubes will not inhibit microbial growth in adjacent tissues and far from the surface. This can be due to not presence of diffusible agents in nano-tubes in Group 2. Therefore, it is necessary to add diffusible antimicrobial agents, such as ions and drugs, to make and increase antimicrobial properties farther away from the mini-implant surface.
ZnO has anti-bacterial properties.[29,35] Its mechanism is to produce reactive oxygen species and destroy the integrity of the cell wall of bacteria.[30] Moreover, the released Zn2+ ions are absorbed by the negatively charged polysaccharide layer in the cell wall of bacteria and disrupt the balance of the membrane and cause bacterial death.[36] Xu et al.[22] showed the antibacterial effect of Zn on S. mutans attached to the surface.[34] Petrini et al.[24] showed that ZnO on titanium surface significantly reduced five streptococcus species.[31] Lui et al.[28] showed that the release of Zn from TiO2 nanotubes can have continuous antibacterial properties against S. mutans and P. gingivalis.[31]
Applications of antibiotics such as amoxicillin, tetracycline, and doxycycline have also been suggested to add antibacterial properties to the surface of implants.[32] Doxycycline has also shown the characteristics of improving bone growth and treatment of periodontal disease as well as periimplantitis.[31,32]
Ferreira et al. showed doxycycline coated on nano-tubes implant surface reduced the growth of P. gingivalis during 1 month.[29]
In the third group of the present study, ZnO and doxycycline were added to titanium nano-tubes. The antimicrobial results are compatible with the above-mentioned studies. The inhibitory zones were formed from the first 5-day to fifth 5-day periods. This means that nano-tubes containing doxycycline and zinc oxide, at least until the 1st month, can inhibit the growth of bacteria on the surface of mini-implants and adjacent tissues. The clinical efficacy of this method and the duration of its effective time should be evaluated in future studies.
P. gingivalis, used in this study, is in the red complex and the most potent species in causing periodontal disease, destroying periodontal support, and inflammation around the implant. It increases in fixed orthodontic treatments and causes significant changes in gingival microbial flora.[14] The type of microbial growth environments, temperature, incubation period, and the required size are expressed by clinical standards (CLSI). Based on this protocol and the mean of inhibitory zones mentioned in it for different bacteria and antibiotics (approximately less than 30 mm), the inhibitory zone observed in the first 5-day period (48 mm), can be suggested that doxycycline and ZnO have a synergistic effect.
CONCLUSION
1. TiO2 nano-tubes do not have an anti-microbial effect on adjacent tissues alone and the presence of diffusible agents together with them is necessary.
The formation of nanotubes on the dental mini implant surface, although having antimicrobial properties for the surface, is ineffective for microbes that are far from the surface of the mini implant and cause inflammation and infection in adjacent tissues.
2. The combined application of ZnO and doxycycline as diffusible agents into TiO2 nano-tubes has an impressive antimicrobial effect. Therefore, it can be suggested as a way to improve the surface of orthodontic mini-implants and promote their success rate.
Declaration of patient consent
Patient consent not required as there are no patients in this study.
Financial support and sponsorship
Nil.
Conflicts of interest
There are no conflicts of interest.
References
- Evaluation of the effects of different surface configurations on stability of miniscrews. Sci World J. 2013;2013:396091.
- [CrossRef] [PubMed] [Google Scholar]
- Evaluation of interdental space of the maxillary posterior area for orthodontic mini-implants with cone-beam computed tomography. Am J Orthod Dentofac Orthop. 2009;135:635-41.
- [CrossRef] [PubMed] [Google Scholar]
- Overview development of orthodontic micro-implants for intraoral anchorage. J Clin Orthod. 2003;37:321-8.
- [Google Scholar]
- Advances in orthodontic anchorage with the use of mini-implant techniques. Br Dent J. 2015;218:E4.
- [CrossRef] [PubMed] [Google Scholar]
- Current products and practice: Bone anchorage devices in orthodontics. J Orthod. 2006;33:288-307.
- [CrossRef] [PubMed] [Google Scholar]
- The use of miniscrew implants for temporary skeletal anchorage in orthodontics: A comprehensive review. Oral Surg Oral Med Oral Pathol Oral Radiol Endodontol. 2007;103:e6-15.
- [CrossRef] [PubMed] [Google Scholar]
- Determinants for success rates of temporary anchorage devices in orthodontics: A meta-analysis (n> 50) Eur J Orthod. 2014;36:303-13.
- [CrossRef] [PubMed] [Google Scholar]
- Failure rates and associated risk factors of orthodontic miniscrew implants: A meta-analysis. Am J Orthod Dentofacial Orthop. 2012;142:577-95.e7.
- [CrossRef] [PubMed] [Google Scholar]
- Effect of surface anodization on stability of orthodontic microimplant. Korean J Orthod. 2012;42:4-10.
- [CrossRef] [PubMed] [Google Scholar]
- Failure rates of mini-implants placed in the infra zygomatic region. Prog Orthod. 2015;16:31.
- [CrossRef] [PubMed] [Google Scholar]
- Microflora associated with successful and failed orthodontic mini-implants. Clin Oral Implants Res. 2009;20:1186-90.
- [CrossRef] [PubMed] [Google Scholar]
- Antibacterial effect of doxycycline-coated dental abutment surfaces. Biomed Mater. 2015;10:55003.
- [CrossRef] [PubMed] [Google Scholar]
- Microbial colonization in orthodontic mini-implants. Braz Dent J. 2012;23:422-7.
- [CrossRef] [PubMed] [Google Scholar]
- Review of titanium surface modification techniques and coatings for antibacterial applications. Acta biomaterialia. 2019;83:37-54.
- [CrossRef] [PubMed] [Google Scholar]
- The role of implant surface characteristics in the healing of bone. Crit Rev Oral Biol Med. 1996;7:329-45.
- [CrossRef] [PubMed] [Google Scholar]
- In vivo histological response to anodized and anodized/hydrothermally treated titanium implants. J Biomed Mater Res Part B Appl Biomater. 2003;66:520-5.
- [CrossRef] [PubMed] [Google Scholar]
- Bone regeneration performance of surface-treated porous titanium. Biomaterials. 2014;35:6172-81.
- [CrossRef] [PubMed] [Google Scholar]
- The surface anodization of titanium dental implants improves blood clot formation followed by osseointegration. Coatings. 2018;8:252.
- [CrossRef] [Google Scholar]
- TiO2 nanotubes functionalized with regions of bone morphogenetic protein-2 increases osteoblast adhesion. J Biomed Mater Res Part A. 2008;84:447-53.
- [CrossRef] [PubMed] [Google Scholar]
- Microstructure and antibacterial property of in situ TiO2 nanotube layers/titanium biocomposites. J Mech Behav Biomed Mater. 2012;8:178-83.
- [CrossRef] [PubMed] [Google Scholar]
- Zinc-ion implanted and deposited titanium surfaces reduce adhesion of Streptococcus mutans. Appl Surf Sci. 2010;256:7540-4.
- [CrossRef] [Google Scholar]
- Review on zinc oxide nanoparticles: Antibacterial activity and toxicity mechanism. Nanomicro Lett. 2015;7:219-42.
- [CrossRef] [PubMed] [Google Scholar]
- Antibacterial activity of zinc modified titanium oxide surface. Int J Artif Organs. 2006;29:434-42.
- [CrossRef] [PubMed] [Google Scholar]
- Bioactive implant surface with electrochemically bound doxycycline promotes bone formation markers in vitro and in vivo. Dent Mater. 2014;30:200-14.
- [CrossRef] [PubMed] [Google Scholar]
- Qualitative and quantitative changes in the oral bacterial flora occur shortly after implementation of fixed orthodontic appliances. Am J Orthod Dentofacial Orthop. 2019;156:735-44.
- [CrossRef] [PubMed] [Google Scholar]
- Preparation of various boron-doped TiO2 nanostructures by in situ anodizing method and investigation of their photoelectrochemical and photocathodic protection properties. J Iran Chem Soc. 2019;16:1839-51.
- [CrossRef] [Google Scholar]
- Synthesis of TiO2 nanotubes with ZnO nanoparticles to achieve antibacterial properties and stem cell compatibility. Nanoscale. 2014;6:9050-62.
- [CrossRef] [PubMed] [Google Scholar]
- Efficiency of nanotube surface-treated dental implants loaded with doxycycline on growth reduction of Porphyromonas gingivalis. Int J Oral Maxillofacial Implants. 2017;32:322-8.
- [CrossRef] [PubMed] [Google Scholar]
- Antibacterial activity and cytocompatibility of an implant coating consisting of TiO2 nanotubes combined with a GL13K antimicrobial peptide. Int J Nanomed. 2017;12:2995-3007.
- [CrossRef] [PubMed] [Google Scholar]
- Sustained co-delivery of ibuprofen and basic fibroblast growth factor by thermosensitive nanoparticle hydrogel as early local treatment of peri-implantitis. Int J Nanomed. 2019;14:1347-58.
- [CrossRef] [PubMed] [Google Scholar]
- Morphologic studies on the biologic seal of titanium dental implants. Report I. In vitro study on the epithelialization mechanism around the dental implant. Int J Oral Maxillofac Implants. 1998;13:457-64.
- [Google Scholar]
- Bacterial composition at the implant-abutment connection under loading in vivo. Clin Implant Dent Relat Res. 2016;18:138-45.
- [CrossRef] [PubMed] [Google Scholar]
- Decreased Staphylococcus aureus biofilm growth on anodized nanotubular titanium and the effect of electrical stimulation. Acta Biomater. 2011;7:3003-12.
- [CrossRef] [PubMed] [Google Scholar]
- Targeted Drug Delivery of Hard Tissue Implants with Bilayer Coating Titanium Oxide Nanotubes and Porous Polymer Nanorods. Medical Engineering and Laboratory Equipment. :46-9.
- [Google Scholar]
- Antimicrobial properties of ZnO nanomaterials: A review. Ceram Int. 2017;43:3940-61.
- [CrossRef] [Google Scholar]
- Electrochemically induced anatase inhibits bacterial colonization on titanium grade 2 and Ti6Al4V alloy for dental and orthopedic devices. Colloids Surf B Biointerfaces. 2011;88:648-55.
- [CrossRef] [PubMed] [Google Scholar]