Translate this page into:
Novel cytotoxicity of nano-coated orthodontic micro-implants: An in vitro study
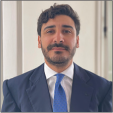
*Corresponding author: Giuseppe Minervini, Multidisciplinary Department of Medical-Surgical and Odontostomatological Specialties, University of Campania “Luigi Vanvitelli”, Naples, Italy. giuseppe.minervini@unicampania.it
-
Received: ,
Accepted: ,
How to cite this article: Othman SS, Albagdaly M, Nahidh M, Hameed MA, Marrapodi M, Cicciù M, et al. Novel cytotoxicity of nano-coated orthodontic micro-implants: An in vitro study. APOS Trends Orthod. doi: 10.25259/APOS_78_2024
Abstract
Objectives:
Many attempts have been made to modify the surface of orthodontic micro-implants and prevent the development of microbes by coating them with antimicrobial nanoparticles (NPs). The purpose of the present study was to evaluate the cytotoxicity of different NPs, namely, TiO2 and zinc oxide (ZnO) NPs, that are used to coat titanium orthodontic micro-implants.
Material and Methods:
Thirty orthodontic micro-implants were included in this study. Those were divided into three groups: control group without coating, TiO2-coated orthodontic micro-implants, and TiO2- and ZnO-coated orthodontic micro-implants. Scanning electron microscope, energy-dispersive spectroscopy (EDS), and cytotoxicity tests were applied for all groups.
Results:
The results of scanning electron microscopy and EDS showed effective deposition of the titanium oxide layer onto the micro-implants. The ZnO layer applied on the micro-implants exhibited superior physicochemical characteristics in comparison to the uncoated samples with no significant cytotoxicity.
Conclusion:
Both NPs showed biocompatibility with the oral tissues.
Keywords
Orthodontics
Anchorage
Anti-microbial
Nanoparticles
Micro-implants
INTRODUCTION
The continued development of orthodontic mini-screws (OMSs) utilized in orthodontic interventions within the past decade has led to a notable transformation in therapeutic viewpoints. The main advantages of dental mini-implants are the guarantee of skeletal anchoring and the promotion of effective long-term orthodontic treatment.[1-3]
Although OMSs have a high success rate in serving as reliable anchorage devices for various malocclusion instances, approximately 30% of failures occur because of bacterial infections and inflammation of the surrounding soft tissues and bones. Furthermore, when significant stresses are applied, there is a tenuous bond between the bone and the surface of the mini-screw.[4]
Various techniques have been developed to enhance the surface properties of orthodontic micro-implants to reduce inflammation and improve their stability. These methods include sandblasting, microgrooving, anodic oxidation, plasma ion implantation, nano-scale modification, and nanoparticle (NP) incorporation.[4] Orthodontic applications have utilized NP-coated arch wires, adhesives, elastomeric ligatures, temporary anchorage devices, orthodontic wires with shape memory and biofilm management properties, as well as nano-metal-coated brackets.[5]
Direct current (DC) magnetron sputtering technology was used to coat the surface of micro-implants with titanium oxide NPs.[6] The application of TiO2 NPs as a coating is anticipated to offer several benefits. The benefits of bio-inert dental materials containing TiO2 NPs are their high flexural strength, biocompatibility, bone-generating characteristics, regenerative potential, and osteogenic potential.[7,8]
Zinc oxide (ZnO) NPs have demonstrated potent antibacterial properties against both Gram-positive and Gram-negative bacteria, as well as spores that are highly resistant to elevated temperatures and pressure.[9,10]
The hypothesized mechanisms for ZnO NPs involve the generation of hydrogen peroxide and the deposition of particles onto the bacteria’s surface through electrostatic interactions. In addition, the antibacterial activity of these NPs could be attributed to various factors, such as the production of reactive oxygen species on the surface of the particles, the release of Zinc ions, disruption of the cell membrane, and the internalization of the NPs.
Cao et al.[11] conducted a review and found that certain authors have reported the presence of cyto-and genotoxicity, but only when the quantities are exceptionally high. Prior investigations conducted did not reveal any cytotoxic or genotoxic effects of TiO2-NPs in primary human cells.[12] Since the oral epithelium is primarily composed of non-keratinized stratified squamous epithelium, save for the gingiva, hard palate, and dorsal side of the tongue, there is a possibility for direct interaction between NPs and the cells and tissues of the oral cavity.[13]
The NPs produced during treatment may result in systemic toxicity or direct damage to the cells and tissues of the oral mucosa. The effects of exposure to TiO2, ZnO2, and Ag NPs have been assessed in multiple in vitro and animal investigations.[14-16]
Prior studies have shown that NPs of titanium dioxide (TiO2) can be taken in by the lungs and gastrointestinal tract.[17] The titanium NPs (Ti NPs) have the potential to be released from the dental implants and enter the surrounding periodontal tissues or freshly formed bone.[18-20]
Based on the previous findings, the objective of the present study was to produce titanium micro-implants modified with TiO2 NPs and titanium micro-implants modified with TiO2 and ZnO NPs. Two distinct approaches were employed for this purpose: analysis and comparison of the cytotoxicity of the altered micro-implants were conducted by evaluating their impact on several cell lines. The null hypothesis stated that coating micro-implants with TiO2 and ZnO NPs has a cytotoxic effect.
MATERIAL AND METHODS
Sample
In the investigation, micro-implants made of titanium alloy (Tomas®, Dentaurum, Inc., Germany) were utilized.
With three groups and all three of these conditions met, the sample size was determined using G power 3.0.10 (a program created by Franz-Faul, University of Kiel, Germany). The sample size was 30 samples (10 samples for each group), with power of study = 80%, alpha error of probability = 0.05 two-sided, and effect size of F = 0.60 (large effect size).[21]
Sample distribution was based on the coating method employed and the specific coating materials utilized, resulting in the division of the micro-implants into three distinct groups.
Experimental group without surface coating.
Titanium oxide (TiO2)-coated group; here, micro-implants were coated with a layer of titanium oxide (TiO2) using DC spattering.
Titanium oxide- and ZnO (TiO2 ZnO)-coated group; here, micro-implants were coated with a titanium oxide layer using DC spattering and a ZnO layer using laser vacuum.
Methods
DC sputtering technique
This work utilized homemade DC-reactive magnetron sputtering equipment. Titanium dioxide (TiO2) thin films were fabricated on glass substrates by sputtering a 99.99% pure titanium target in the presence of oxygen.
Before introducing a 1:1 combination of Argon and Oxygen gases into the deposition chamber, the chamber was evacuated to a pressure of 0.001 mbar. The discharge current was 20 milli-amperes, while the pressure of the gas mixture was around 0.8 millibar.
To initiate plasma-based ion etching, the micro-implants were positioned within a vacuum chamber and the chamber was subsequently filled with Argon gas. The plasma discharge is generated through the process of gas heating, which leads to ionization. Consequently, the plasma that is formed contains positively charged gas ions and negatively charged electrons. Subsequently, the substrate is submerged in the plasma under a negative voltage, thereby drawing the energized ions and propelling them toward the substrate at considerable velocities. Ions impact the substrate and disturb the atomic-level arrangement of its surface, resulting in the ejection of particles from the surface until they clash with another surface inside the vacuum environment. This approach generates a unique surface topography at the micro- and nano-sizes.[6]
Laser vacuum technique
In the vacuum plasma production procedure, multiple peak strengths of Nd: YAG laser (Hua Fei Tong Da Technology-Diamond-288 Pattern EPLS) were used.
Model of a laser-equipped with a Q-switch Nd: YAG laser undergoing first harmonic generation. The laser energy range is between 100 and 400 millijoules (mJ).
Wavelengths of the laser: 1064 and 532 nm – The repetition rate is 6 Hz.
Input for AC power supply with a voltage of 220 Volts.
The power supply facilitates the light path system in the form of a portable external device. The object’s measurements are 25 cm in length, 21 cm in width, and 38 cm in height. It weighs 16 kg. The pulse energy can be incremented in 100 millijoule increments. The laser spot size can be adjusted by varying the distance between the laser lens and the target. The number of pulses can be controlled and varied. However, the duration of each laser pulse remains constant at 9 nanoseconds. The compression of ZnO NPs yielded round pellets of 3 cm in diameter and 2 mm in thickness.
The chamber was emptied by employing a two-stage rotary pump from CITALCATEL (Annecy, France) until the pressure reached a base level of 210-2 Torr.
A convex spherical quartz lens, with a focal length of 10 cm, was employed to concentrate the YAG laser onto the target surface. The lens was positioned in front of the vacuum chamber. Precise laser focusing is necessary to surpass the energy density threshold required for ablation when the laser makes contact with the surface of the target material.
Characterization of the surface coating on the titanium micro-implants
Scanning electron microscopy (SEM)
Electron microscopy (SEM, Carl Zeiss Microscopy Deutschland Gm, Oberkochen, Germany) and energy-dispersive spectroscopy (EDS) were used to examine and visualize the surface morphology of the three groups of micro-implants.
Coating particles’ atomic interactions with electrons provide surface topography and composition images. Very high-resolution pictures of the coating particles’ surfaces can be obtained using SEM, which is useful for comprehending the sample’s surface structure. When electrons are scattered or emitted from the surface of the samples that have been manufactured, a detector is used to gather them. Over a 300 × 700 nm region, survey scan analyses were performed.
Cytotoxicity tests
MMT (3-(4, 5-dimethylthiazolyl-2)-2, 5-diphenyltetrazolium bromide) assay of cytotoxicity
Mixed human osteocytes and osteoblast cell line (MG-63), ATCC: (CRL-1427) product code number representing Mixed human osteocytes and osteoblast cell, normal human lung fibroblast cell line (WI0-38, [CCL-75] product code number representing normal human lung fibroblast cell line), and normal human gingival fibroblasts cells (HGF1-PI) product code number representing human normal gingival cells, ATCC: CRL-2014 were kindly provided by the International Center for Training and Advanced Researches, Cairo, Egypt. Cells were grown in MEM-E medium supplemented with 10% fetal bovine serum (GIBCO, Thermo Fisher Scientific, Waltham, MA, USA) at 37°C in a humidified 5% CO2 atmosphere (Jouan SA, Saint-Herblain, Pays de la Loire, France).
Cells were maintained in accordance with the manufacturing protocol, which included decanting the growth medium and washing the cells with phosphate buffer saline (PBS) (Adwia Pharmaceuticals, Sharqia, Egypt). Cells were treated for 5 min at 37°C with 0.25% trypsin enzyme and 0.05% (v/v) ethylenediaminetetraacetic acid (GIBCO). Separated cells were spat out in accordance with need.
Cytotoxicity was performed according to Senthilraja and Kathiresan [22] in which MG63, WI-38, and HGF1-PI cell lines were propagated in 75 cm2 cell culture ([TPP]-Swiss Techno Plastic Products, Switzerland). Cells were plated at a density of 2 × 105 cells/mL in 96-well cell culture plates and incubated at 37°C for 24 h to achieve confluence. The growth medium was decanted, and fresh medium with serially diluted titanium samples was added to pre-cultured plates. Dead cells were washed out 24 h later with (PBS, pH = 7.2 0.2 [Adwia], and 50 l of MTT (3-(4,5-dimethylthiazol-2-yl)-2,5-diphenyltetrazolium bromide) stock solution (0.5 mg/mL) was added per well.
The supernatant was discarded after 4 h of incubation at 37°C, and the formazan precipitate was solubilized by adding 50 l/well of dimethyl sulfoxide. Plates were incubated in the dark for 30 min at 37°C, and absorbance was measured at 570 nm using a microplate reader (ELx-800, Bio-Tek Instruments, Inc., Winooski, VT, USA). The percentage of cell viability was calculated as follows: The viability percentage is calculated as the mean optical density (OD) of the test dilution divided by the mean OD of the control wells.
Extraction of test materials
Titanium-precoated metals were weighed using a digital balance (Sartorius, Germany). Metal samples were steam-sterilized at 121°C for 20 min (CISA-Italy). E-MEM medium (GIBCO-USA) was added to sterilized metals as 3 mL total volume for 14 days. The extraction medium was harvested and sterile-filtered using a disposable syringe filer (Millipore-USA).
RESULTS
SEM results
Controlled group
[Figure 1] displays scanning electron micrographs of micro-implants made of titanium alloy. A flawless titanium coating uniformly covers the micro-surface implant’s topography. Emerging structures with typical lateral diameters of 400– 500 nm make up the coating’s non-directional homogenous structure.
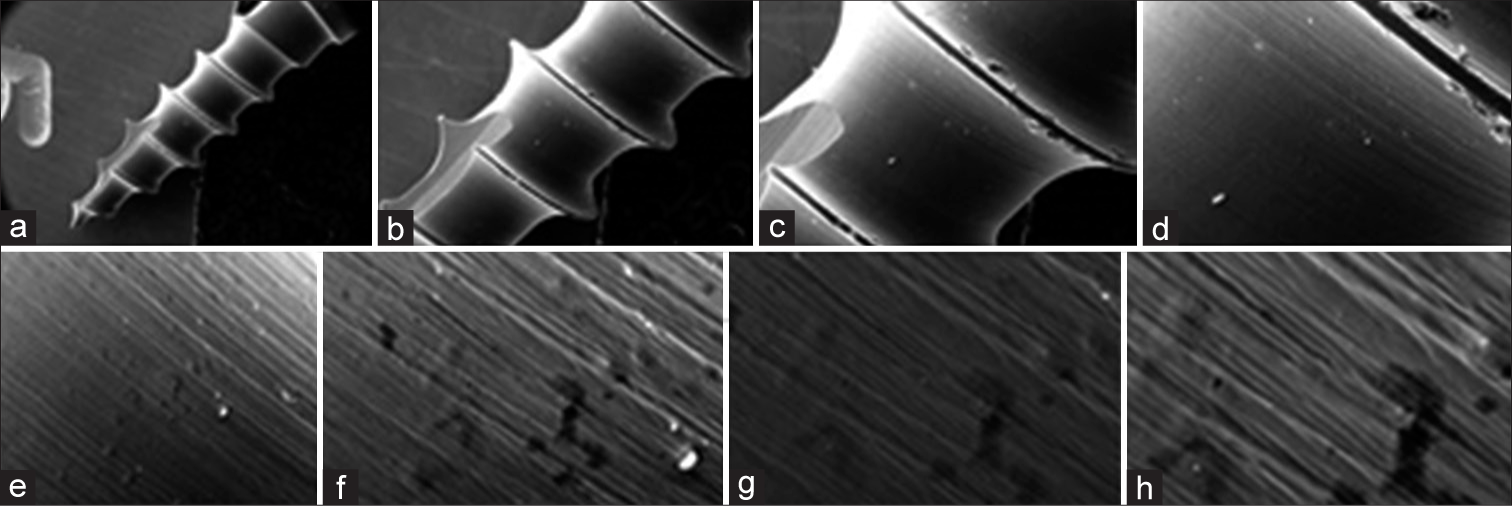
- Scanning electron microscopy images of titanium alloy (Ti6Al4V) micro-implants at magnifications of (a) 61×. (b) 130×. (c) 250×. (d) 500×. (e) 1000×. (f) 2000×. (g) 3000× and (h) 4000×.
[Figure 2] shows the EDS spectra of SEM images of uncoated micro-implants. The peaks in the EDS spectra that correspond to titanium and oxygen confirmed that they were 94% Ti and 6% C, respectively. The elemental composition of the materials was validated by EDS analysis, which qualitatively confirmed this.
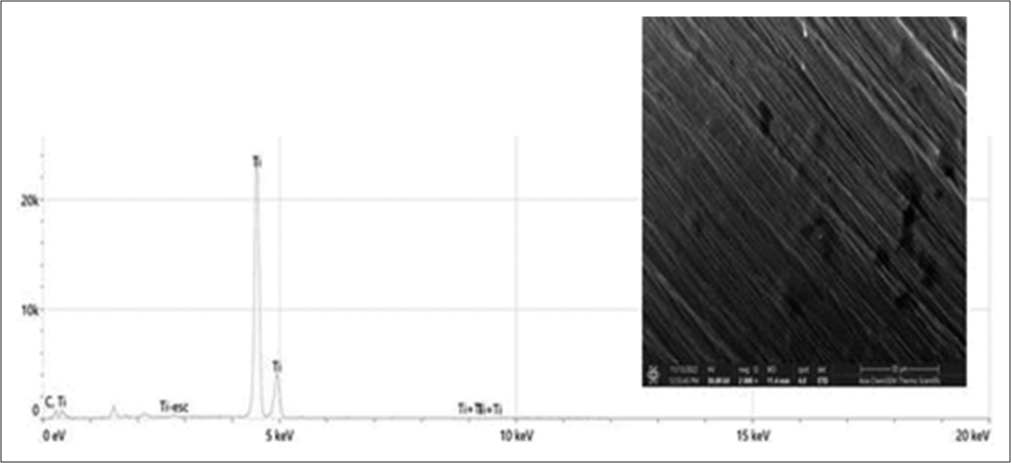
- The energy-dispersive spectroscopy spectrum of Field Emission-Scanning electron microscopy images for uncoated micro-implants.
TiO2-coated group
The scanning electron microscope images of the micro-implants, which were coated with a titanium oxide layer using the DC magnetron sputtering method, revealed an elliptical black region in the middle. This indicates that the coating completely covered the titanium surface of the implant, and there was a neighboring region rich in titanium. One may witness high uniformity, spherical shape of NPs, and absence of particle clustering. [Figure 3] displays a homogeneous crystalline surface characterized by a compact structure, numerous depressions, restricted areas, and visible pores.
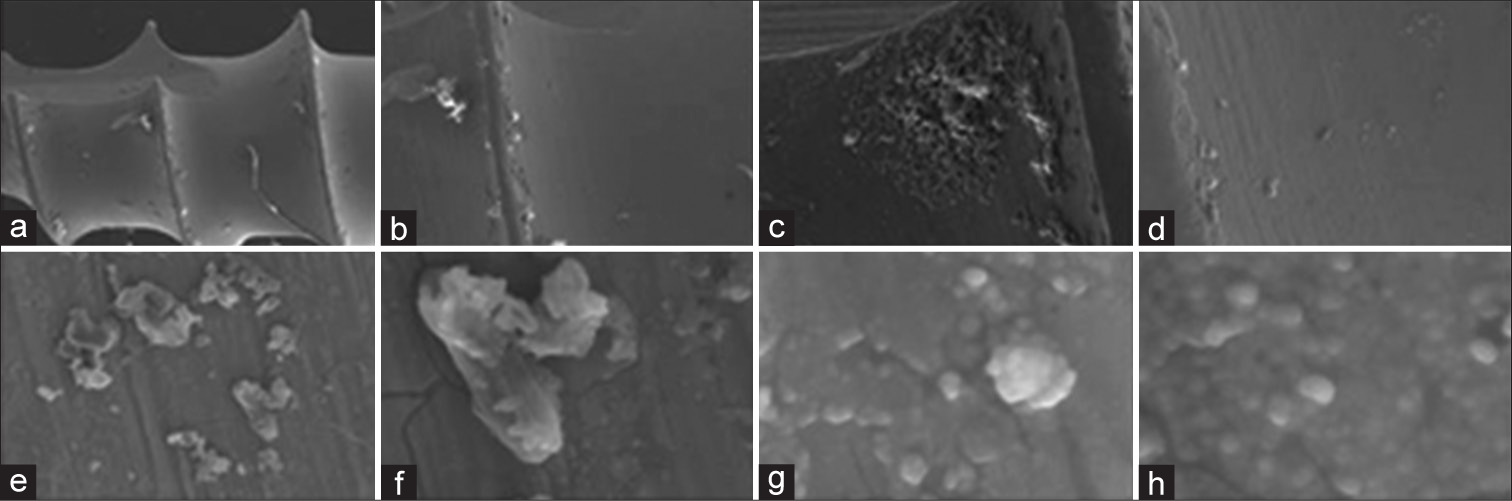
- Scanning electron microscopy images of titanium alloy (Ti6Al4V) micro-implants coated with titanium oxide layer by direct current magnetron spattering method at magnifications of (a) 50×. (b) 150×; (c) 300×. (d) 500×. (e) 3.00K×. (f) 10.00K×. (g) 25.00× and (h) 35.00K×.
Conversely, the chemical composition determined by EDS analysis revealed that the sample consisted of 19.68% oxygen and 80.32% titanium. The results demonstrated the effective deposition of the titanium oxide layer onto the micro-implants, which was confirmed using EDS analysis, as depicted in [Figure 4].
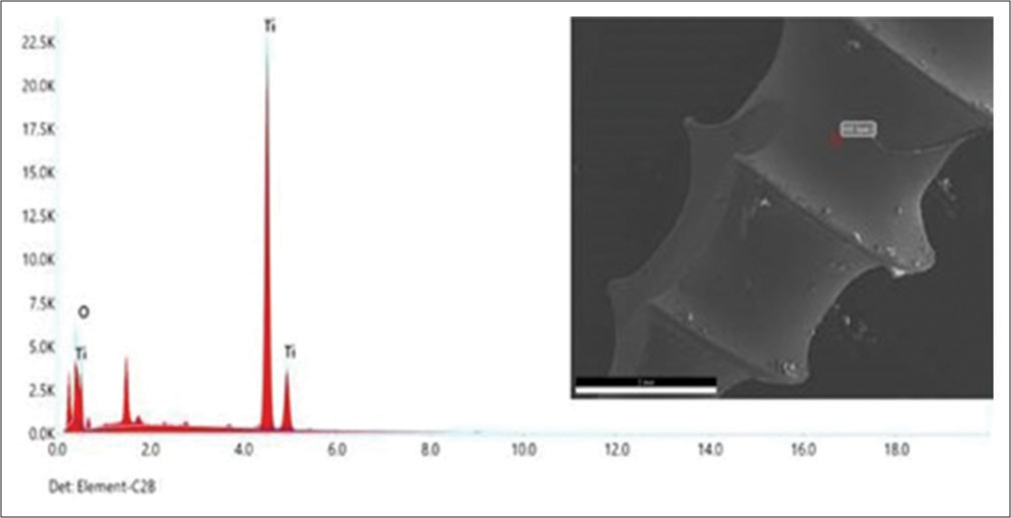
- The energy-dispersive spectroscopy spectrum of FE-scanning electron microscopy images for titanium alloy (Ti6Al4V) micro-implants coated with titanium oxide layer by direct current magnetron spattering method.
TiO2- and ZnO-coated group
SEM pictures in [Figure 5] depict TiO2: ZnO NP micro-implants with a scattered layer of mixed nano-oxides of different thicknesses covering the whole surface of the micro-implant. The surface of the micro-implant also exhibits a matrix with both mesoporous and macroporous characteristics. None of the samples contained any agglomerates or clumps. The surface of the exterior morphology exhibits prominent grooves resulting from the application of Ti NPs during the coating process. The compact oxide coating serves to inhibit the release of ions from the metallic substrate of the micro-implants. The high deposition rate of the ZnO coating in the rich zone leads to an increased surface area on the sample.
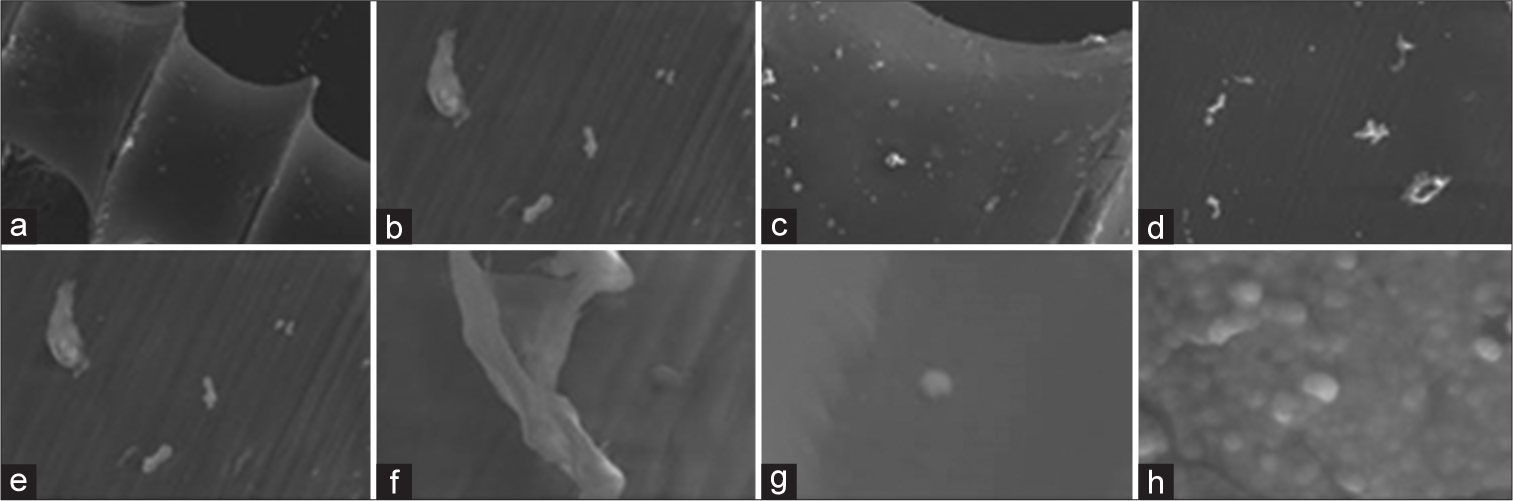
- Scanning electron microscopy images of titanium alloy (Ti6Al4V) micro-implants coated with titanium oxide layer by direct current spattering method and another layer of zinc oxide layer by laser vacuum method at magnifications of (a) 50×. (b) 100×. (c) 150×. (d) 500K×. (e) 1.00K×. (f) 3.00K×. (g) 25.00× and (h) 35.00K×.
[Figure 6] illustrates the EDS spectrum of TiO2: ZnO NP micro-implants. Based on the EDS studies, the products were primarily composed of Zn, Ti, and O, which accounted for 1.85%, 95.09%, and 3.06% of the total weight, respectively. According to the EDS analysis, the Zn element was incorporated into the coatings by the laser vacuum technique.
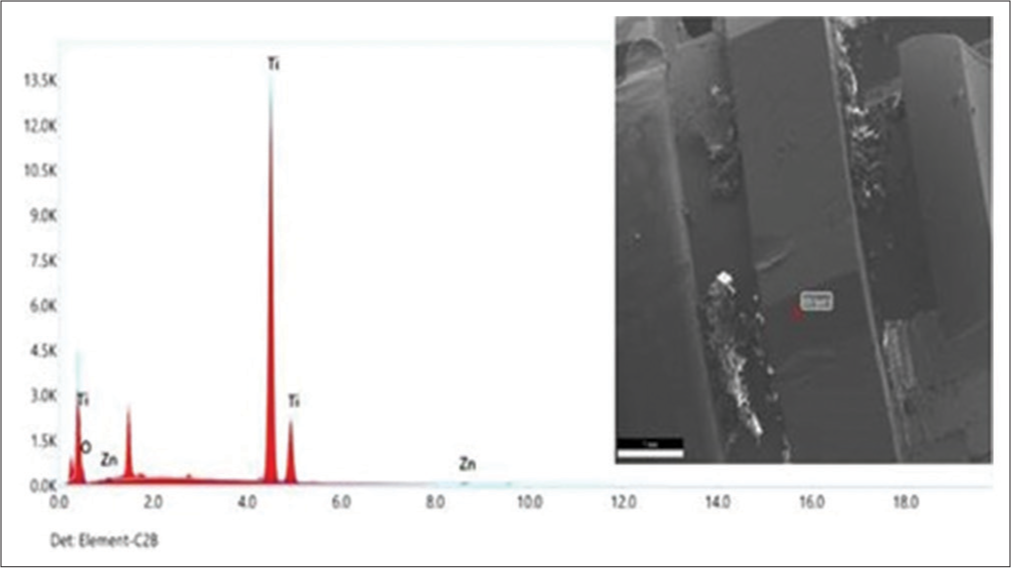
- The energy-dispersive spectroscopy spectrum of FE-Scanning electron microscopy images for titanium alloy (Ti6Al4V) micro-implants coated with titanium oxide layer by direct current spattering method and another layer of zinc oxide layer by laser vacuum method.
Cytotoxicity evaluation of Zn ONPs- and TiO2NPs-coated micro-implants
The cytotoxicity was also tested at several concentrations for both ZnO NPs and TiO2 NPs against normal human osteocytes MG-63, fibroblasts WI-38, and gingival tissue HGF1-PI. Results in [Figures 7-9] explain that no significant toxicity was found against three types of cells. The cytotoxicity results confirmed that the coated materials showed less toxicity to the host cell.
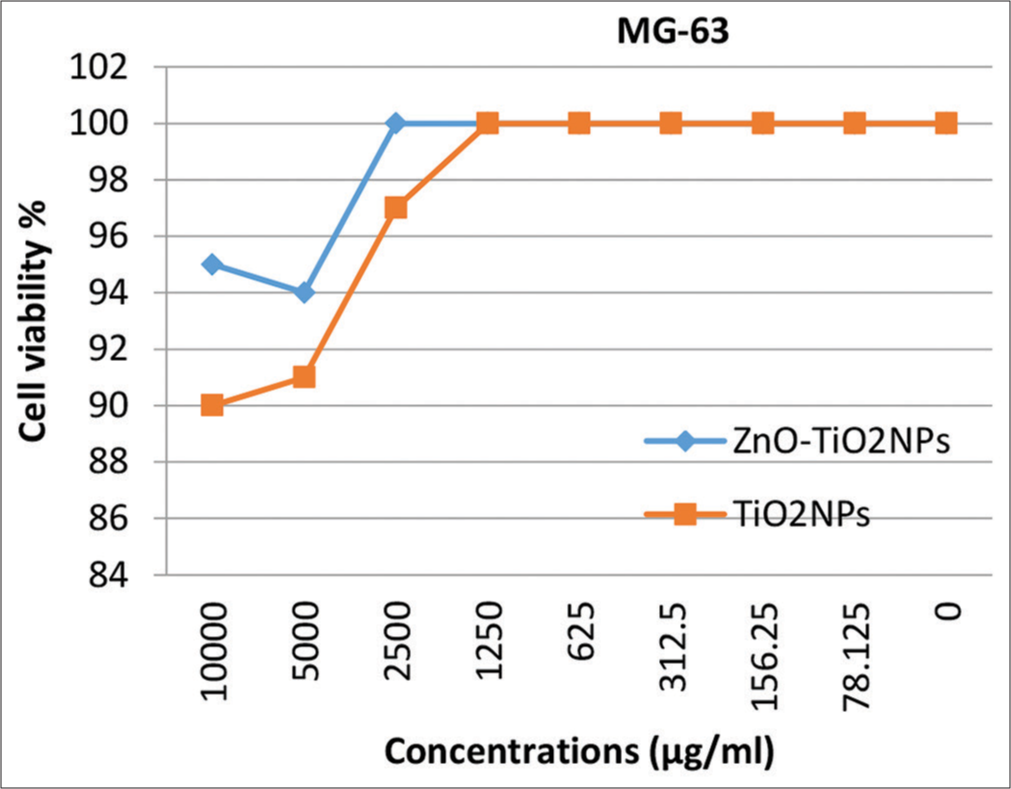
- Cytotoxicity of zinc oxide nanoparticles (NPs) and TiO2NPs on osteocytes MG-63. MG-63: Mixed human osteocytes and osteoblast cell line, ZnO-TiO2 NP: Zinc oxide-titanium oxide nano particles; TiO2 NP: Titanium oxide nano particles.
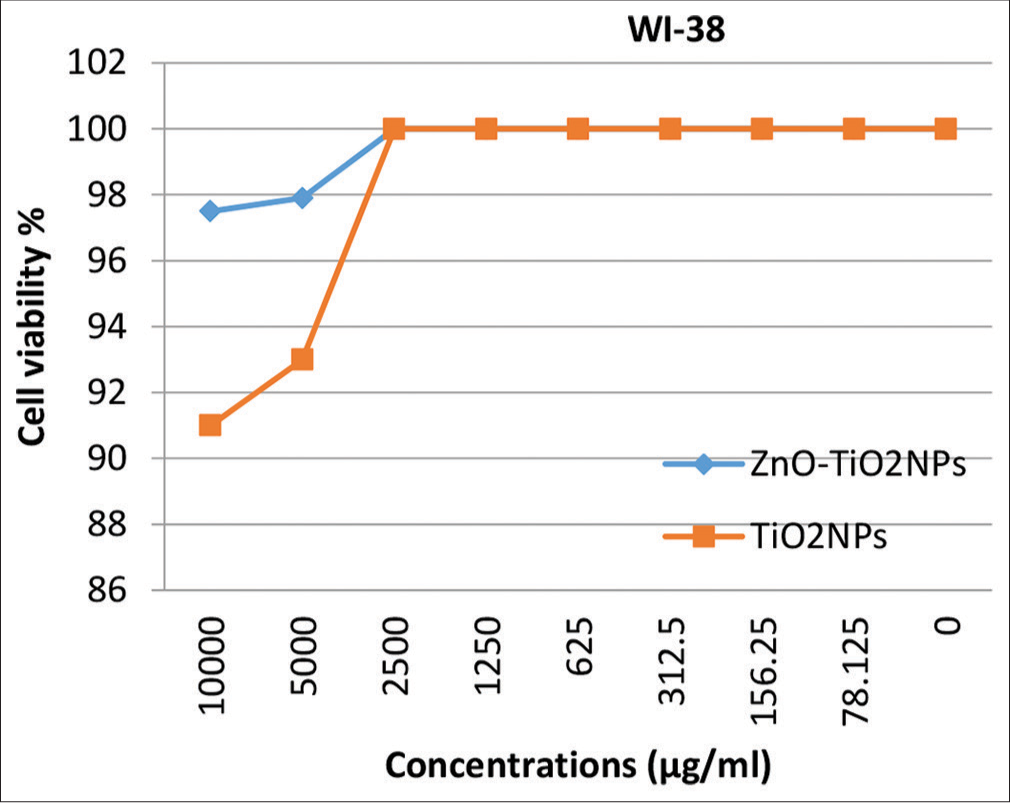
- Cytotoxicity of zinc oxide nanoparticles (NPs) and TiO2NPs on fibroblasts WI-10. W10: Normal human lung fibroblast cell linel; ZnO-TiO2 NP: Zinc oxide-titanium oxide nano particles; TiO2 NP: Titanium oxide nano particles.
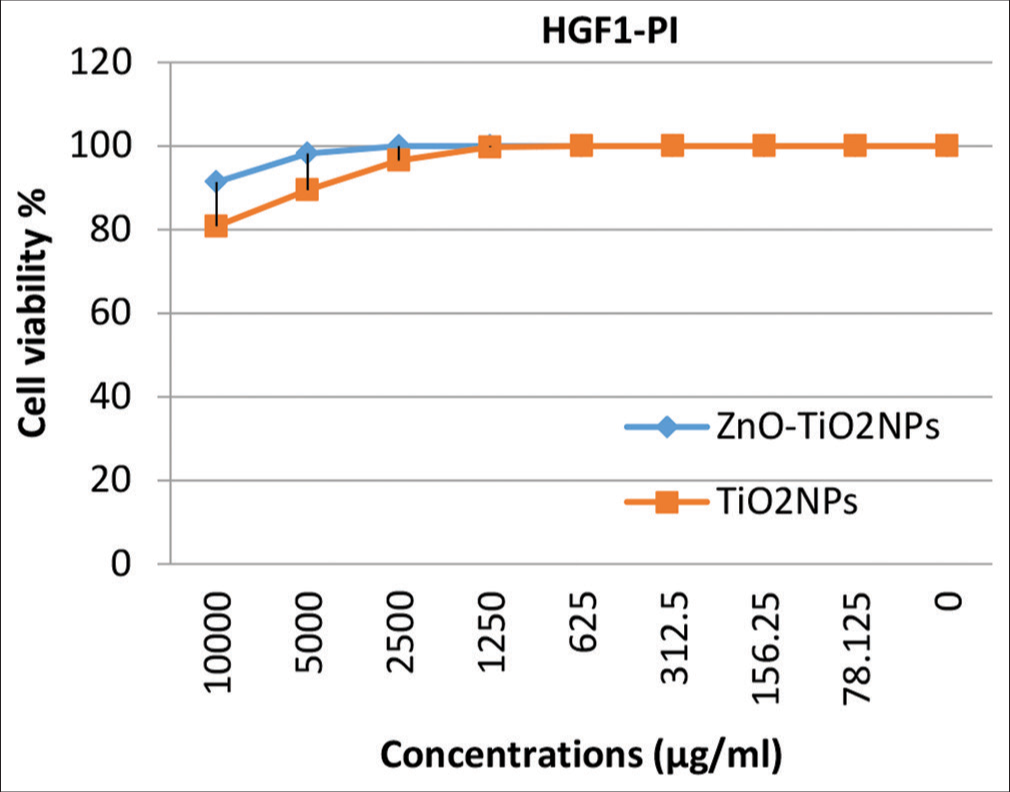
- Cytotoxicity of zinc oxide nanoparticles (NPs) and TiO2NPs on gingival tissue human gingival fibroblasts 1-PI-38. HGF1: Human normal gingival cells, ZnO-TiO2 NP: Zinc oxide-titanium oxide nano particles; TiO2 NP: Titanium oxide nano particles.
DISCUSSION
Medicine and dentistry utilize a range of nano-particles. TiO2 NPs, which are readily accessible in many dimensions and crystalline configurations, are highly suitable for integration into dental materials. They have a low cost and possess excellent mechanical properties, as well as an appealing type.[23,24] In response to conflicting findings on potential negative health impacts on the human body, the present study investigates the deposition, aggregation, and cytotoxic effects of TiO2 and ZnO-NPs on human cells.
Since the oral epithelium is primarily composed of nonkeratinized stratified squamous epithelium and protects the gingiva, hard palate, and dorsal side of the tongue, there is a possibility for direct interaction between NPs and the cells and tissues of the oral cavity.[13] The Ti NPs have the potential to be discharged from the dental implants and enter the nearby periodontal tissues or freshly formed bone.[18-20] For this reason, mixed human osteocytes and osteoblast cells, normal human lung fibroblasts, and normal human gingival cell lines were tested for cytotoxic reactions in response to orthodontic micro-implants coated with TiO2 NPs and TiO2 with ZnO NPs in the present study.
Prior studies have shown that NPs of TiO2 can be taken up by the lungs and gastrointestinal tract.[17] However, studies conducted on live rodents demonstrated heightened deposition of nano-metals from dental materials in the internal organs, potentially leading to organ pathology. For example, administering TiO2 NPs (25 or 80 nm) through oral gavage resulted in pathological alterations in the liver and kidney of mice. Similarly, long-term consumption of colloidal silver solution led to the development of argyria in people.[25,26]
The results of the present study showed high cell viability rates even with high concentrations of TiO2 NPs which are not standing with the results of the studies mentioned above. These results are in coincidence with the results of previous studies.[12,14,27] While other studies considered TiO2 NPs to be generally regarded as non-cytotoxic, the inclusion of these NPs at a concentration of 1% w/w in orthodontic adhesive resulted in a moderate level of cytotoxicity on HGF1.[15,28]
ZnO-NPs are a significant category of nano-materials commonly used in diagnostics, therapies, medication delivery, personal care products, and food additives.[29,30] Nano-particulate ZnO exhibits superior UV-protective properties compared to bulky ZnO powder while also avoiding the drawbacks of being occlusive and white.
There have been ongoing concerns throughout the past decade regarding the toxicity of ZnO-NPs. Multiple researchers consistently documented the ability of ZnO-NPs to cause genetic damage, either in laboratory experiments or in living organisms.[31-35]
However, the characteristic finding in the presented study was the use of a combined coating of TiO2 and ZnO NPs; the MTT assay showed that the cell viability of the three cell lines used was higher with this mixture than with TiO2 alone.
The best explanation of this finding is that the disintegration of ZnO nanoparticles and the attachment of the released Zn2+ ions onto TiO2 nanoparticles through adsorption will decrease the cytotoxic effects of TiO2 nanoparticles. Considering the findings of the present investigation, this could serve as an explanatory framework for the reduced genotoxicity of ZnO-NPs when combined with TiO2-NPs. The collective application appears to have little effect on the cytotoxicity of ZnO-NPs. However, it does considerably decrease DNA damage and promote regeneration.[36]
These results coincided with the findings of Hackenberg et al.[27], who concluded that a synergistic strategy utilizing both TiO2-NPs and ZnO-NPs concurrently could be regarded as a more secure choice in comparison to solely employing ZnONPs. While there is limited evidence in the scientific literature suggesting that TiO2-NPs can be harmful at very high doses, combining them with ZnO-NPs may enable the use of lower quantities for both substances, resulting in safer outcomes.
The SEM photographs of the micro-implants in the TiO2-coated group revealed the presence of an elliptical black region in the middle. This observation indicates that the coating completely covered the titanium surface of the implant, with a neighboring region rich in titanium. One may witness high uniformity, spherical shape of NPs, and absence of particle clustering. Similarly, there is a consistent, highly structured crystalline surface that has numerous small depressions, enclosed areas, and visible pores. Unlike the control group, the experimental group exhibited a uniformly textured surface with no fissures and a non-directional, homogeneous structure. The obtained results were similar to those of prior investigations.[37,38] Conversely, the luminous regions shown in SEM pictures of titanium oxide- and ZnO-coated micro-implants are a result of the rapid deposition of the ZnO coating in the concentrated area. The rough sample’s exterior morphology exhibits prominent surface grooves resulting from the application of a titanium NP coating technique. Within these primary grooves, there are also smaller grooves present. This results in an increased surface area of the sample. Moreover, the compact oxide layer serves to inhibit the release of ions from the metallic base of the micro-implants. The controlled group micro-implants exhibit distinct peaks in their EDS patterns that correspond to Ti, and O. The C peak is a result of C pollutants that have been adsorbed onto the surface, which is exposed to the ambient environment. This is a common occurrence with any material before being inserted into the SEM vacuum system.
The EDS patterns of TiO2-coated micro-implants exhibit transition energies of 4.508 keV and 4.93 keV, corresponding to Ti and O2, respectively. The energy transition elements for O2 are 0.5 keV, indicating the presence of TiO2 coating on the surface of the micro-implant, which aligns with a previous study.[39-45] In contrast, the EDS examinations of the group coated with TiO2 ZnO reveal that Zn, Ti, and O accounted for most of the products, with weight percentages of 1.85%, 95.09%, and 3.06%, respectively. Zn was incorporated into the coatings by the laser vacuum technique.
The combination of ZnO and TiO2 results in the formation of a composite chemical layer, acting as a thin bonding layer, which is distinctly visible between the ZnO and TiO2 layers. The laser vacuum approach of depositing the ZnO layer was discovered to result in an augmentation of the specific surface area at the nano-scale. In addition, the ZnO layer applied to the micro-implants exhibited superior physio-chemical characteristics in comparison to the uncoated samples. While certain tests have indicated the transfer of electrolyte species into the laser vacuum coating, the exact mechanism remains unidentified. The inclusion of Zn as an active metal element can be a complex process including ion electro-migration, adsorption, and diffusion. Further research is required to gain a comprehensive understanding of the associated challenges.
This study reveals that coating micro-implants with TiO2 ZnO NPs have the advantage of improving the surface of these implants with the antimicrobial effect of these NPs without affecting biocompatibility.
The limited sample size, the absence of a scratch test, and the need for genotoxicity tests and long-term investigation to inspect how these nano-coatings affect the surrounding tissues and the micro-implants were the limitations of the present study, which require further research in future studies.
CONCLUSION
Based on the obtained results, the following conclusions could be drawn:
NP deposition on the titanium surface of micro-implants allows for the design of morphological and structural features on the nanoscale.
The cytotoxic results revealed the biocompatibility of these NPs with the oral tissues, so the null hypothesis was rejected. However, more animal research is needed to investigate their efficiency in clinical orthodontics.
Ethical approval
The Institutional Review Board approval is not required.
Declaration of patient consent
Patient’s consent was not required as there are no patients in this study.
Conflicts of interest
There are no conflicts of interest.
Use of artificial intelligence (AI)-assisted technology for manuscript preparation
The authors confirm that there was no use of artificial intelligence (AI)-assisted technology for assisting in the writing or editing of the manuscript and no images were manipulated using AI.
Financial support and sponsorship
Nil.
References
- Use of temporary anchorage devices in orthodontics: A review of the literature. Web Med Cent Orthod. 2013;4:WMC004458.
- [Google Scholar]
- Early treatment of class II division 1 malocclusions with prefabricated myofunctional appliances: A case report. Prosthesis. 2023;5:1049-59.
- [CrossRef] [Google Scholar]
- Impacted central incisors in the upper jaw in an adolescent patient: Orthodontic-surgical treatment-a case report. Appl Sci. 2022;12:2657.
- [CrossRef] [Google Scholar]
- Clinical protocol for implant-assisted partial removable dental prostheses in kennedy class I: A case report. Prosthesis. 2023;5:1002-10.
- [CrossRef] [Google Scholar]
- Antimicrobial efficacy of silver, zinc oxide, and titanium dioxide nanoparticles incorporated in orthodontic bonding agent. J Bagh Coll Dent. 2019;31:10-16.
- [CrossRef] [Google Scholar]
- Nanoparticles deposition on mini-implants for osseo-integration and antibacterial properties improvement. Rev Chim. 2017;68:2929-31.
- [CrossRef] [Google Scholar]
- An experimental method to add new prosthetic teeth in the removable partial denture framework: TIG cold welding and preformed pins. Prosthesis. 2023;5:1120-8.
- [CrossRef] [Google Scholar]
- Development of nanoparticles for antimicrobial drug delivery. Curr Med Chem. 2010;17:585-94.
- [CrossRef] [Google Scholar]
- Post-traumatic stress, prevalence of temporomandibular disorders in war veterans: Systematic review with meta-analysis. J Oral Rehabil. 2023;50:1101-9.
- [CrossRef] [Google Scholar]
- Comparison of the effect of mouthwashes with and without zinc and fluocinolone on the healing process of erosive oral lichen planus. J Dent Res Dent Clin Dent Prospect. 2010;4:25-8.
- [Google Scholar]
- Genotoxicity evaluation of titanium dioxide nanoparticles in vivo and in vitro: A meta-analysis. Toxics. 2023;11:882.
- [CrossRef] [Google Scholar]
- Color stability, physical properties and antifungal effects of ZrO2 additions to experimental maxillofacial silicones: Comparisons with TiO2. Prosthesis. 2023;5:916-938.
- [CrossRef] [Google Scholar]
- Conservative treatment of temporomandibular joint condylar fractures: A systematic review conducted according to PRISMA guidelines and the cochrane handbook for systematic reviews of interventions. J Oral Rehabil. 2023;50:886-93.
- [CrossRef] [Google Scholar]
- In vitro cytotoxicity assessment of an orthodontic composite containing titanium-dioxide nano-particles. J Dent Res Dent Clin Dent Prospects. 2013;7:192-8.
- [Google Scholar]
- In vitro cytotoxicity of maxillofacial silicone elastomers: Effect of nano-particles. J Prosthodont. 2018;27:584-7.
- [CrossRef] [Google Scholar]
- Effect of eluates from zirconia-modified glass ionomer cements on DNA double-stranded breaks in human gingival fibroblast cells. Dent Mater. 2019;35:444-9.
- [CrossRef] [Google Scholar]
- Titanium dioxide nanoparticles: A review of current toxicological data. Part Fibre Toxicol. 2013;10:15.
- [CrossRef] [Google Scholar]
- Early detachment of titanium particles from various different surfaces of endosseous dental implants. Biomaterials. 2004;25:2239-46.
- [CrossRef] [Google Scholar]
- Mapping of titanium particles in peri-implant oral mucosa by laser ablation inductively coupled plasma mass spectrometry and high-resolution optical darkfield microscopy. J Oral Pathol Med. 2011;40:412-20.
- [CrossRef] [Google Scholar]
- Corrosion of metal orthopaedic implants. J Bone Joint Surg Am. 1998;80:268-82.
- [CrossRef] [Google Scholar]
- Statistical power analysis for the behavioral sciences (2nd ed). Hillsdale, NJ: Erlbaum; 1988.
- [Google Scholar]
- In vitro cytotoxicity MTT assay in vero, HepG2 and MCF-7 cell lines study of marine yeast. J Appl Pharm Sci. 2015;5:80-4.
- [CrossRef] [Google Scholar]
- Improving performance of dental resins by adding titanium dioxide nanoparticles. Dent Mater. 2011;27:972-82.
- [CrossRef] [Google Scholar]
- Nanoparticle-reinforced resin based dental composites. J Dent. 2008;36:450-5.
- [CrossRef] [Google Scholar]
- Acute toxicity and biodistribution of different sized titanium dioxide particles in mice after oral administration. Toxicol Lett. 2007;168:176-85.
- [CrossRef] [Google Scholar]
- Silver products for medical indications: Risk-benefit assessment. J Toxicol Clin Toxicol. 1996;34:119-26.
- [CrossRef] [Google Scholar]
- Genotoxic effects of zinc oxide nanoparticles in nasal mucosa cells are antagonized by titanium dioxide nanoparticles. Mutat Res Genet Toxicol Environ Mutagen. 2017;816-7:32-7.
- [CrossRef] [Google Scholar]
- Effects of TiO2 nano glass ionomer cements against normal and cancer oral cells. In Vivo. 2014;28:895-907.
- [Google Scholar]
- Exposure to ZnO nanoparticles induces oxidative stress and cytotoxicity in human colon carcinoma cells. Toxicol Appl Pharmacol. 2010;246:116-27.
- [CrossRef] [Google Scholar]
- Zinc oxide nanostructures: Synthesis and properties. J Nanosci Nanotechnol. 2005;5:1561-73.
- [CrossRef] [Google Scholar]
- Effectiveness of adjunctive hyaluronic acid application in surgical treatment of gingival recession sites. Prosthesis. 2023;5:635-46.
- [CrossRef] [Google Scholar]
- Clastogenicity, photo-clastogenicity or pseudo-photo-clastogenicity: Genotoxic effects of zinc oxide in the dark, in pre-irradiated or simultaneously irradiated Chinese hamster ovary cells. Mutat Res. 2006;607:215-24.
- [CrossRef] [Google Scholar]
- Repeated dose dermal toxicity study of nano zinc oxide with Sprague-Dawley rats. Cutan Ocul Toxicol. 2012;31:26-32.
- [CrossRef] [Google Scholar]
- Acute toxicity of ferric oxide and zinc oxide nanoparticles in rats. J Nanosci Nanotechnol. 2010;10:8617-24.
- [CrossRef] [Google Scholar]
- Acute toxicity of zinc oxide nanoparticles to the rat olfactory system after intranasal instillation. J Appl Toxicol. 2013;33:1079-88.
- [CrossRef] [Google Scholar]
- Prevalence of temporomandibular disorders (TMD) in obesity patients: A systematic review and meta-analysis. J Oral Rehabil. 2023;50:1544-53.
- [CrossRef] [Google Scholar]
- Silane-based coating charged with TiO2 NPs for dental implant applications. Surf Coatings Technol. 2021;413:127066.
- [CrossRef] [Google Scholar]
- Do titanium mini-implants have the same quality of finishing and degree of contamination before and after different manipulations? An in vitro study. Metals (Basel). 2021;11:245.
- [CrossRef] [Google Scholar]
- Facile synthesis of highly thermally stable TiO2 photocatalysts. New J Chem. 2017;41:5021-7.
- [CrossRef] [Google Scholar]
- Outstanding, yet redundant? after all, you may be another choluteca bridge! Semin Orthod. 2021;27:53-6.
- [CrossRef] [Google Scholar]
- The effect of vestibular, lingual, and aligner appliances on the quality of life of adult patients during the initial stages of orthodontic treatment. Prog Orthod. 2021;22:3.
- [CrossRef] [Google Scholar]
- Short-term cone-beam computed tomography evaluation of maxillary third molar changes after total arch distalization in adolescents. Am J Orthod Dentofac Orthop. 2019;155:191-7.
- [CrossRef] [Google Scholar]
- Accuracy of digital predictions with CAD/CAM labial and lingual appliances: A retrospective cohort study. Semin Orthod. 2018;24:393-406.
- [CrossRef] [Google Scholar]
- Artificial intelligence (AI) driven orthodontic care: A quest toward utopia? Semin Orthod. 2021;27:57-61.
- [CrossRef] [Google Scholar]
- Assessing accelerated tooth movement techniques on their own catabolic merits: A review. J World Fed Orthod. 2018;7:122-7.
- [CrossRef] [Google Scholar]