Translate this page into:
Effects of three types of attachments in the displacements, and distribution of stress and plastic deformation, and trough finite elements (FEM)

*Corresponding author: Valentina Fayad Jaramillo, Department of Orthodontics, Fundación Universitaria CIEOUniCIEO, Bogota, Colombia. vfayadj@gmail.com
-
Received: ,
Accepted: ,
How to cite this article: Melo Andrade WA, Fayad Bajaire WE, Fayad Jaramillo V, González García TA. Effects of three types of attachments in the displacements, and distribution of stress and plastic deformation, and trough finite elements (FEM). APOS Trends Orthod. 2024;14:48-56. doi: 10.25259/APOS_19_2023
Abstract
Objectives:
The aim of the study was to evaluate the effect of resin attachments on the displacement and deformation pattern of the aligner plastic, determine the center of rotation and stress distribution in the upper canine during space closure using the finite element method (FEM), and evaluate the plastic Aligner deformation.
Material and Methods:
A computer-assisted design model of the superior right hemiarch was constructed with the simulation of a first premolar extraction and a canine distalization, recreating the periodontal ligament and alveolar bone. FEMs were created to analyze the behavior of stress and displacement of the upper right canine with aligners in four situations, one without attachment, and three with bonded attachments (vertical rectangular, rectangular beveled, optimized root control). In addition, the plastic deformation of the aligner was evaluated.
Results:
In the simulation without attachment, there was no apical movement, while with vertical rectangular and rectangular beveled the behavior was similar with crown distal displacement and mesial apex displacement. The optimized attachment had a better movement control of the canine body respecting the crown and apical movement toward the same direction. The mayor plastic deformation was shown without attachment and no deformation was evidenced with optimized attachment.
Conclusion:
The FEM showed that nevertheless the optimized attachment produced a distal crown and apical displacement with the use of plastic aligners during the space closure, the apex only moved ¼ of the distal crown movement. Our results suggest that to obtain a pure translation of the canine with aligners, it would be necessary the use of auxiliars which complement the needed biomechanics.
Keywords
Finite element analysis
Orthodontic space closure
Orthodontic appliances
Removable
Dental aligners
Orthodontics
Finite Element Analysis
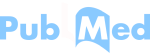
INTRODUCTION
The use of aligners began in 1990 with their introduction by Align Technology[1] in the U.S. They were created to treat simple malocclusions and relapses. Several studies have shown, however, that they could also be used for complex orthodontic cases that had been believed to be treatable only with fixed appliances, such as premolar extractions. This innovative orthodontic technique is widely accepted because it is considerably more esthetic than braces, both metallic and ceramic, besides promoting oral hygiene and substantially reducing the number of emergency appointments needed with traditional orthodontic appliances.[1-3]
Initially, aligners were based only on an empirical system in which the biomechanics of the aligners, the forces generated in the teeth, and their surrounding tissues were unknown. When aligners became more and more popular, they were investigated worldwide, as they were one of the newest and less explored knowledge fields in orthodontics. Diverse systematic reviews of literature (SRLs)[1,4,5] have evaluated the efficacy of clear aligners for different tooth movements and for space closure in extraction cases, but no conclusion could be made based on solid scientific evidence as few clinical studies have been found on this topic. Papadimitriou et al.[5] found limited efficacy of clear aligners for bodily tooth movement and extraction space closure. In the comparison by Li et al.[6] of the effectiveness of the Invisalign aligner with that of braces in extraction cases using the ABO model grading system, the braces had significantly superior objective grading system (OGS) scores for correcting buccolingual inclination and occlusal contacts. However, the OGS scores of the braces and Invisalign for marginal ridges and root angulation were similar. Womack[7] presented a case of four premolar extractions treated with Invisalign using 5 mm long, 2 mm wide, and 1 mm thick rectangular canine attachments that were recontoured into a “ski slope” shape to allow for easier removal of the plastic. Womack reported that in the case refinement phase, he kept the final aligners in place and added power arms gingival to the upper and lower canines as well as buttons to the molars for the attachment of elastics, to parallelize the roots adjacent to the closed space.
Different attachments have been recommended by clear aligner systems to control the distal movement of the canine in space closure cases. Rectangular attachments were first used, but optimized root control attachments were introduced later to improve root control during the distalization movement of the canine.[8,9] However, most clinical studies did not specify what type of attachment they used in extraction cases. Some studies with finite element methods (FEMs)[10,11] compared different attachment shapes and their effects on the distal movement of the canine to the extraction space with clear aligners. Gomez et al.[10] constructed a FEM of the distal movement of an upper canine with plastic aligners with an optimized root control attachment and found bodily movement with the optimized root control attachment and the uncontrolled tipping attachment. However, one of the limitations of this study was its basic single-tooth model. Comba et al.,[11] in a FEM study, found that the rectangular attachment caused buccal displacement of the tooth and the optimized attachments produced bodily translation but with some intrusion, which is why they recommended adding a 4-oz Class II elastic in the upper canine.
Analysis with FEM simulation is an effective tool because it is non-invasive, precise, and can simulate the human biology with various biomechanics in conditions that are very similar to those in the oral cavity. Thus, it allows for the study of homogenous samples while controlling all the variables[12,13] and shows detailed quantitative data on the responses in the periodontal ligament (PDL), alveolar bone, and dental movement during the extraction space closure.[2,14] Movements such as translation, which requires radicular control, and the rotation of teeth with a round shape, such as the canine and premolars, normally require the use of attachments to facilitate such movements. This study evaluates the distribution of displacements, the rotation center, and the distribution of stress in the upper right canine during space closure with an aligner with and without attachments through FEM) and evaluates the plastic aligner deformation.
MATERIAL AND METHODS
The digital FEM model was built using cone-bean computerized tomography (CBCT) of a 35-year-old woman. The CBCT was previously taken for diagnosis of an orthodontic condition. The study meets the ethical principles in the Declaration of Helsinki[15] and was approved by the ethics committee of UniCIEO with ethical guarantee 121, act 64.
The CBCT was taken using a Planmeca dental tomography equipment (Planmeca OY, Helsinki, Finland) with equal vertical and horizontal widths of 0.4 mm. The images were archived in the DICOM format and overlapped with an STL archive that corresponded to intraoral scanning in Blue Sky Plan software (Pmdi Europa GmbH, Langenhagener, Germany). Then, the upper maxillary was segmented and exported as an STL archive to simulate the PDL and the alveolar bone, and the information for building the sectioned dental arch was obtained from an STL archive of the maxillary arch of the patient.
The geometry of the teeth, alveolar bone, and PDL were built using a semi-automatized process, with Mesh Mixer (Autodesk, Inc., San Rafael, CA) simulating the extraction of the first upper right premolar [Figure 1]. The PDL was established as 0.2 mm thick, and its geometry was modeled by detecting the interface area between the bone and the tooth models, because the thickness of the CBCT slice was similar to or even greater than the ligament space, which was approximately 0.2 mm. Each cut of the 3D model in the simulation was delimited by each tooth, and the shape of the teeth for the alveolar bone model was excluded and replaced by segmented teeth to manipulate each tooth within the orthodontic model. All the components of the model were established as isotropic materials and their properties were taken from the previous studies.[10]
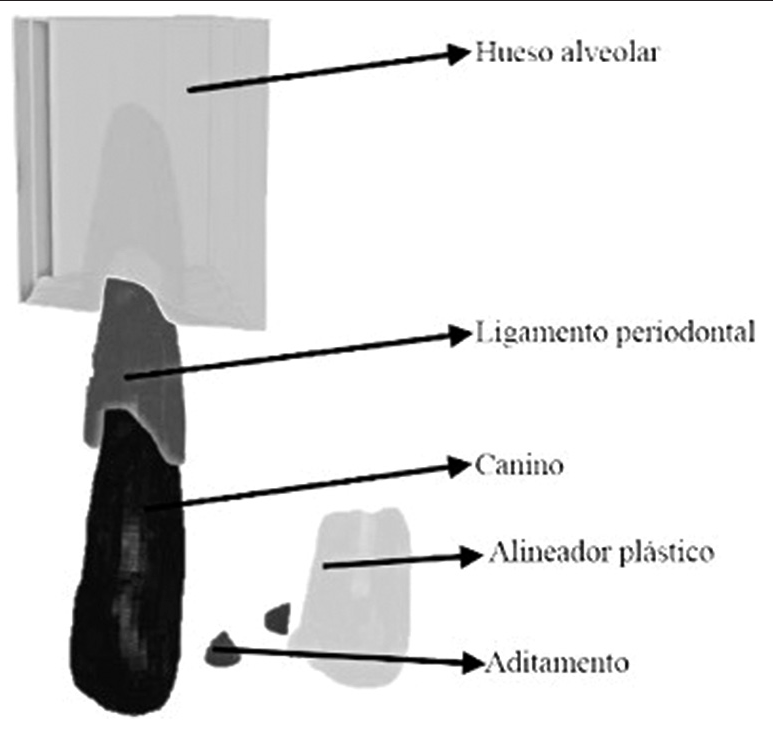
- Geometry of the model created using computer-assisted design.
The aligner was designed with a 0.38-mm thickness based on Align Technology data,[16] creating approximately 0.2 mm of distalization, taken as an isotropic material. The biomodel was designed with the program Mesh (Autodesk, Inc., San Rafael, CA) and included the occlusal surface from tooth 17 to tooth 27, with a scalloped cut 2 mm below the gingival margin.
The distal displacement of the upper right canine was modeled in four situations: Without an attachment, with a rectangular vertical attachment, with a beveled horizontal attachment, and with an optimized attachment. The space closure was simulated at 0.25-mm increments to extrapolate the movement for total space closure, assuming an absolute anchorage for teeth 15, 16, and 17 with an aligner change every 15 days. The extraction space was made virtually. The bonded attachments — rectangular vertical [4.0 mm long × 1.3 mm wide × 0.8 mm high; Figure 2a and b], rectangular beveled [4.0 mm long × 1.37 mm wide at the base × 0.72 mm wide in front × 0.76 mm high × 33° inclination; Figure 3a and b], and optimized root control [2.0 mm high × 2.0 mm wide × 1.5 deep, 1.42-mm diameter; [Figure 4a-c]—were placed in the upper right canine and modeled with the Rhinoceros software (Rhinoceros 3D, McNeel and Associates, Seattle, WA) located in the dental crown, according to a previous study.[2]
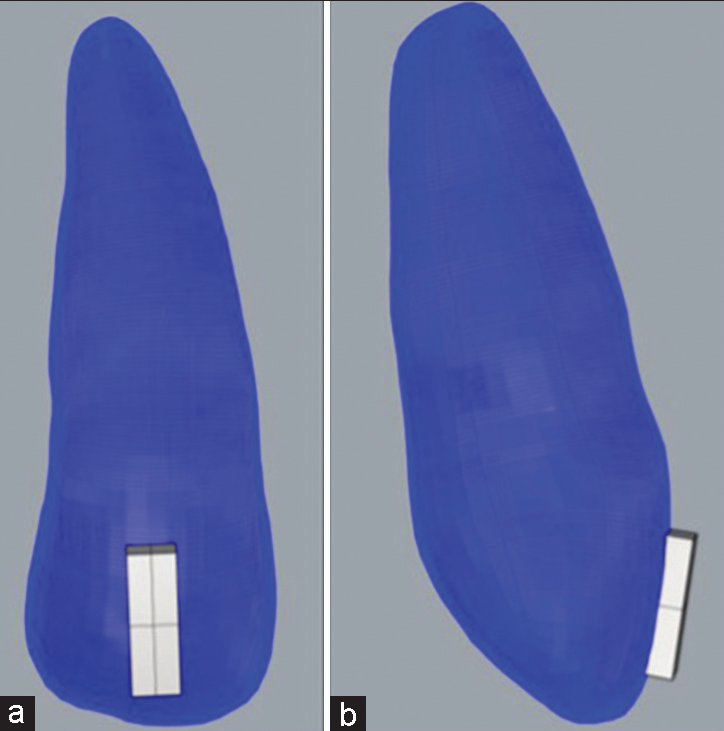
- (a and b) Canine with vertical rectangular attachments.
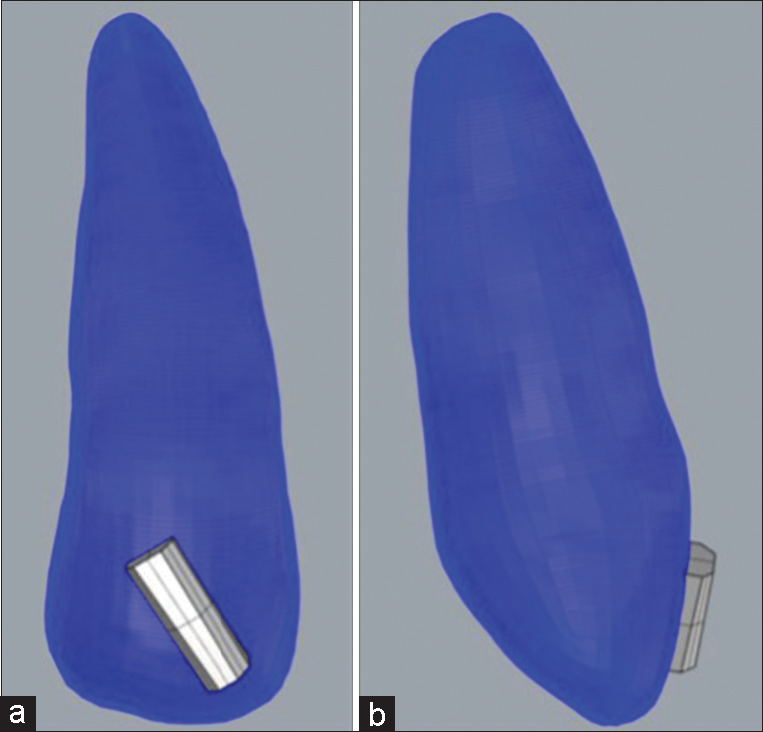
- (a and b) Canine with rectangular horizontal attachment.

- (a-c) Canine with optimized attachment.
All the attachments were placed in the upper right canine and the four simulations were created using Rhinoceros software. The analyzed variables were the distal displacement of the canine on the X axis (i.e., distalization), the trajectory of which was from the initial position of the upper right canine to its final position, to know the magnitude of the first upper-right premolar extraction space closure with the chosen attachments for the simulation; the stress, which is the force exerted on a surface area of a body; and the plastic deformation, which is when a body does not recover its original form after being exposed to a certain force. The center of resistance was calculated based on the bone measurements of the CBCT, taking as a reference the middle apical third. For the fabrication of the FEM, all the components were imported into the Rhinoceros software. The MESH generation was performed using two types of elements: SOLID 187 elements for the volumetric geometries (of the canine, alveolar bone, and attachments) and SHELL 181 elements for the surface geometries (of the PDL and aligner). The resulting volumetric model had 218,594 elements and 318,988 nodes. [Table 1] shows the numbers of elements and nodes used in each structure. The simulation considered all components as isotropic materials using a linear elastic model. [Table 2] shows the properties of the materials, which were taken from a previous study.[10]
Elements | Nodes | Young’s module (MPa) | Poisson’s ratio | |
---|---|---|---|---|
Alveolar bone | 125255 | 182980 | 1.37×103 | 0.30 |
Periodontal ligament | 3802 | 5554 | 1.96×104 | 0.30 |
Canine | 85731 | 125240 | 300 | 0.30 |
Plastic aligner | 3054 | 4462 | 12.5×104 | 0.36 |
Attachment | 508 | 752 | 528 | 0.36 |
FEM: Finite element method
Young’s module (Mpa) | Poisson’s ratio | |
---|---|---|
Bone | 1.37×103 | 0.30 |
Canine | 1.96×104 | 0.30 |
Periodontal Ligament | 30 | 0.30 |
Attachment | 1.25×104 | 0.36 |
Plastic aligner | 528 | 0.36 |
FEM: Finite element method
The distal movement of the upper right canine was modeled in four situations: Without an attachment and with a rectangular vertical attachment, a rectangular beveled attachment, and an optimized root control attachment. The closure of the extraction space was simulated in increments of 0.25 mm,[3] assuming an absolute anchorage and an aligner change every 15 days. The extraction space was created virtually.
In the FEM, the displacement of the canine on the X axis, the stress on the canine, and the deformation of the plastic were measured, regarding the anchorage the maximum. The stress was measured by the maximum principal stress in MPa, since the studied structures were considered non-ductile materials. The displacement and deformation were measured in mm. The center of resistance was calculated according to the bone height measured in the CBCT, taking as a reference the middle third at the root level.
Simulations
Dental movement of the upper right canine with an aligner and no attachment [Figure 5a and b]
Dental movement of the upper right canine with an aligner and a bonded rectangular vertical attachment [Figure 2a and b]
Dental movement of the upper right canine with an aligner and bonded rectangular beveled attachment [Figure 3a and b]
Dental movement of the upper right canine with an aligner and optimized root control attachment [Figure 4a-c].
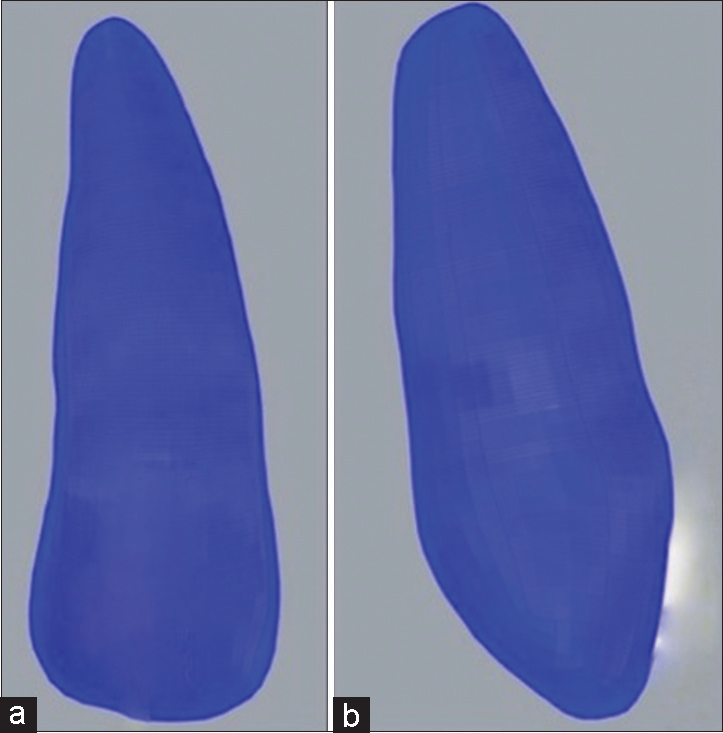
- (a and b) Canine without attachment.
In addition, to verify the reliability of the results, the sensitivity of the meshes was analyzed, and Mesh 4 was selected for its mechanical–structural response.
In the previous studies,[2,17] the contact conditions were established as guaranteed contacts and frictionless contacts. Between the aligner and the tooth, the most important contact surface was represented, which was responsible for the magnitude of the load, and it was designed without friction. The teeth and the PDL were joined by guaranteed contact, and the bonded contacts between the bone and the PDL were considered. A bonded contact corresponded to perfect adhesion between contact surfaces to the corresponding nodes that could not be separated from each other. The bony limbs would be fixed in all directions.
In the present study, we sought to recreate the biological conditions of a patient as closely as possible.
Contact conditions
Alveolar bone – PDL: Guaranteed
PDL – Canine: Guaranteed
Canine – Aligner: Frictionless
Canine – Attachment: Guaranteed
Aligner – Attachment: Frictionless.
The deformation of the plastic was not represented graphically because it directly affected the movement of the canine. It was represented instead as to the stress figures.
RESULTS
Dental movement of the aligner without an attachment
This analysis showed a 0.038-mm distal displacement of the canine crown in each activation without radicular movement. The rotation center was near the medial radicular third. The stress on the canine during the simulation showed a major force on the mesial zone of the PDL in the rotation direction. The deformation of the aligner was 0.6 mm. The simulation showed that without an attachment, there was a slight movement without control and deformation of the aligner [Figure 6a and b].

- (a) Deformation without attachment. (b) Stress without attachment.
Dental movement of the aligner with a bonded rectangular vertical attachment
This analysis revealed greater control of the space closure movement of the extraction space compared to the simulation without an attachment, achieving a maximum distalization of 0.23 mm. The rotation center appeared to be located almost in the apical root center. There was a radicular tip of 0.44 mm in the direction opposite that of the coronal displacement. The stress was concentrated over the rough geometry changes of the attachment, although its distribution in the tooth was more even through the tooth. The deformation of the aligner was 0.27 mm [Figure 7a and b].

- (a) Deformation with vertical rectangular attachment. (b) Stress with vertical rectangular attachment.
Dental movement of the aligner with the bonded rectangular beveled attachment
The deformation given by this simulation revealed a controlled movement of 0.24 mm of the crown and 0.041 mm of the root in the direction opposite that of the coronal displacement, showing a response similar to that with the vertical attachment. The rotation center was almost located in the apical radicular center. The stress that resulted from this simulation showed that the beveled form of the attachment favors the non-appearance of high concentrations of stress in the teeth or the attachment. The aligner deformation was 0.027 mm [Figure 8a and b].
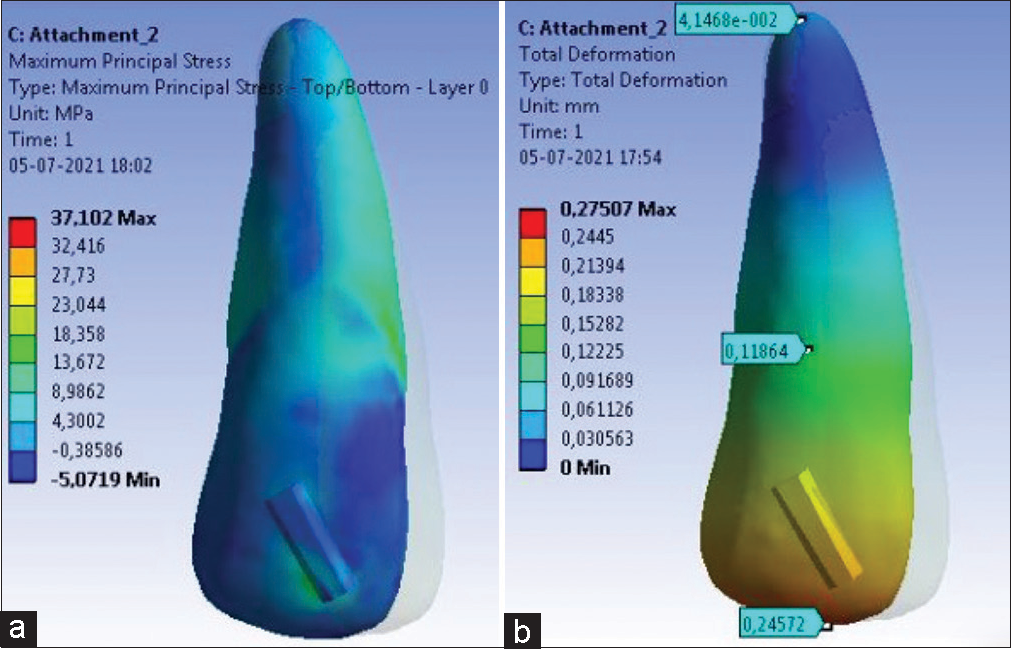
- (a) Deformation, (b) Stress.
Dental movement of the aligner with an optimized root control attachment
This simulation exhibited major control of the other simulations — that is, total movement control that achieved full canine distalizations of 0.22 mm and 0.05 mm of the root in the same direction as that of the coronal displacement. These were obtained by the opposing forces generated with the double attachment that coerced the root and the crown of the canine to move toward the same direction. The rotation center was located at the same position as that in the last simulation. The comparison of the initial position of the canine with its final position revealed that both the crown and the root were displaced distally. Nonetheless, the radicular displacement was greater than that of the crown. The stress in this simulation was distributed equally and generally throughout the teeth and the attachments, and the deformation of the aligner was of 0 mm, showing complete control [Figure 9a and b].
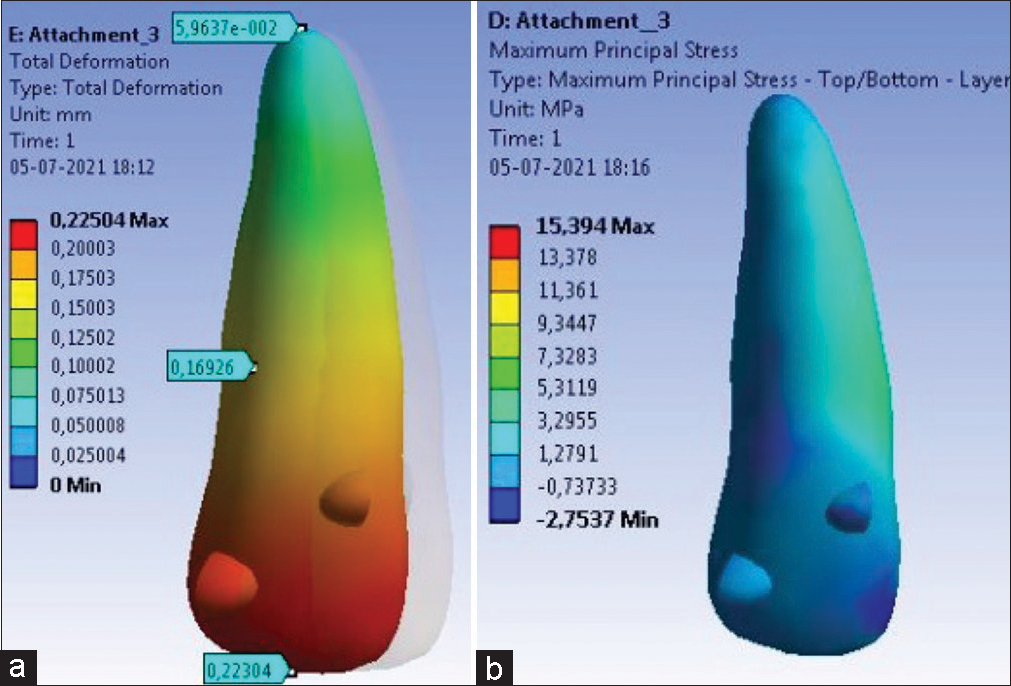
- (a) Deformation with optimized attachment, (b) Stress with optimized attachment.
DISCUSSION
The use of aligners for dental movement has been increasing and it is becoming an excellent option for patients who seek more esthetic orthodontic options. Nevertheless, the effectivity of the dental movement with aligners in complex cases, such as extraction cases, depends on factors such as the attachments and auxiliary movements.[4,18-20] The shapes and sizes of the attachments are very important because they help generate the desired biomechanics and control the unwanted effects that could be generated during the dental movement with aligners.[2,10,11,14,21] The present study analyzed the displacement, stress distribution, and location of the center of rotation in the upper right canine, as well as the plastic aligner deformation, while simulating space closure with three attachments and without an attachment.
The results of our study [Table 3]showed differences in the coronal and apical displacements of the canine and with the center of rotation. While the coronal displacement of the canine was lower in the model without attachments, its values in the other models with attachments were similar. However, the movements at the apical level were different: They were mesial apical movements in the models without an attachment, a bonded rectangular vertical attachment, and a bonded rectangular beveled attachment; and distal apical movement in the optimized root control attachment model. These suggest uncontrolled tilt tooth movement in the models without an attachment and with rectangular and beveled attachments, and more controlled movement close to a body movement in the model with an optimized root control attachment. Likewise, the resistance centers found were consistent with the observed displacements, being in the middle third of the root in the model without an attachment and close to the root apical center in the models with a rectangular vertical attachment, a rectangular beveled attachment, and an optimized root control attachment.
Model | Coronal displacement (mm) | Apex displacement (mm) | Center of rotation | Stress | Plastic deformation (mm) |
---|---|---|---|---|---|
No attachment | 0.038 distal | 0.0 | Apical middle third | More stress in the mesial zone of the Periodontal ligament in the rotation direction | 0.6 |
Vertical rectangular attachment | 0.23 distal | 0.44 mesial | Near the apical middle third | Mayor concentration of stress where the changes of the attachment geometry are abrupt | 0.27 |
Beveled rectangular attachment | 0.24 distal | 0.041 mesial | Near the apical middle third | No high stress concentrations were observed, nor in the attachment of the tooth. | 0.27 |
Optimized attachment | 0.22 distal | 0.05 distal | Near the apical middle third | Soft and even distribution of stress in the tooth and the attachment. | 0 |
Similar results have been found by different researchers[12,22,23] in FEM studies, considering that the optimized attachment is that which provides the greatest biomechanical advantages in closing spaces with aligners. On the contrary, Ho et al.,[24] in a study on resin-printed typodonts in which they evaluated ellipsoid attachments such as the optimized thin and thick and bar attachments, found that the aligner material had a greater influence on the tooth movement of the body than did the shape and size of the attachments.
Although the stress distribution observed in the different simulations in our study were similar to those found by other authors with FEM, their models were slightly different. Gomez et al.[10] realized four FEM models with and without attachments and Class II elastics, which significantly reduced the canine intrusion. Xu et al.[25] created three models with attachments, power arms, and temporary anchorage device (TADs), where the model with power arms resulted in a major and more efficient distalization of the canine. Likewise, our study found that even though the optimized attachment showed better movement control, the distal coronal displacement was greater than the displacement of the distal movement of the apex. This could lead to the failure of the canine to move completely with its body in the end, after the closure of the spaces, which could explain why the complete expression of the mesodistal tip programmed in the aligners is not always achieved. Therefore, the use of auxiliaries such as mini-implants, power arms, and planning aligners in staged movements[10,11,25] is many times necessary to avoid undesired effects in the space closure with aligners.
Regarding our findings on the deformation of the plastic aligner, greater deformation was observed in the simulation without an attachment; lower deformations in the rectangular vertical attachment and the beveled horizonal attachment; and no deformation in the optimized attachment. Gomez Arango et al., Comba et al.[2,11] agreed that some sectors of the aligner are deformed as a result of the forces applied to them and that these deformations can generate undesired movements during treatment with aligners, such as dental intrusion in space closure cases. The present study showed that by not using attachments and using rectangular vertical and beveled horizontal attachments, the aligner was deformed and generated unwanted movement of the canine, such as closure of the space by inclination and not by full body translation, in the simulation with an optimized attachment. Such movement showed the influence of the geometry of the attachment on the deformation. However, this bares a limitation of the present study because the interaction between the change in the attachments and the plastic deformation was not determined. It is very important to consider that the plastic materials used for the manufacture of aligners varied in thickness and type of plastic. In the present study, a 0.75-mm polyurethane aligner was manufactured with a 2-mm cut in the gingival margin to make it more stable and more flexible, and to enable it to yield more predictable results. Iliadi et al.[19] found that the thicknesses of the aligner, 0.5 mm, 0.625 mm, and 0.75 mm, were not related to significant differences in the moment/force ratio with an aligner with a gingival margin of 3–4 mm undercut. As in the present study, Cortona et al.[14] compared the deformation of the aligner with different combinations of attachments in a specific movement. They found that the maximum deformation of the aligner was 0.25 mm. The present study had a similar result for the rectangular vertical and beveled horizontal attachments, with a maximum aligner deformation of 0.27 mm, but total control of the said variable was achieved with the optimized attachment with a deformation of 0 mm.
The clinical predictability of body tooth movements with aligners has been evaluated in various clinical studies and SRLs. Lombardo et al.[26] observed a lack of precision of the M-D movement of the canine between 0.6° and 5°. Cortona et al.[14] found in an SRL that the accuracy of the M-D tilting movements of the maxillary canines was between 39% and 43%. Likewise, Iliadi et al.,[19] in their SRL, found three studies that indicated that the body movement and the torque movement were the most demanding movements to achieve because the aligners alone were not capable of delivering the required system of forces. Therefore, it would be a good idea to add a 5–10-° overcorrection of the mesial coronal tip in the canines in space closures with aligners, as recommended by Mehta et al.[27]
This study could help clinicians to provide reliable information regarding the biomechanical behavior of different attachments when it comes to closing extraction spaces using aligners. Moreover, this study could provide clinicians with higher confidence and assurance when using aligners because their advantages and disadvantages, as well as their limitations, are known variables. However, this does not mean that the research is over, as there are still many more grounds to cover and discover in this field. The entrance of technology into stages such as diagnostics, planification, and treatment is becoming more enriching and is making information more trustworthy every day.
As a disclaimer, it is highly recommended that the results of this study be treated with caution because they came merely from a simulation with FEM of a clinical in vivo situation. In addition, in the present study, all the components of the model were considered isotropic materials, but various authors[11,28,29] mentioned that the PDL should be modeled as a viscoelastic material to obtain results that are closer to clinical reality, since this characteristic of viscoelasticity would allow knowing with greater certainty the real cushioning reaction that occurs during orthodontic movements, especially during the closure of extraction spaces in vivo. This dissipation of forces includes a rearrangement of structural changes in the collagen fibers that are vital to allowing tooth movement. Different authors[22,24,30-33] mentioned the importance of simulating complete models with all the structures that compose the system (the teeth, PDL, and alveolar bone); and although this study is close to reality, it could be not as exact as the actual clinical situation. This means that, in full, the FEM simulations cannot be contrasted with an individual biological response, which can only be attained through clinical trials. Therefore, we recommend that future investigations be made in vivo, based on studies such as this that allow us to know, with a higher level of certainty and confidence, the clinical result of the closure of extraction spaces in premolars with the use of dental aligners. In such studies, the reaction of the teeth and adjacent tissue can be observed with precision, as well as the plastic deformation and the adaptation process throughout the entire movement over the X and Y axes.
CONCLUSION
Although the use of attachments generates greater control in the distal movement of the canine, compared to the absence of attachments during the space closure with aligners, only the optimized attachment produced a distal movement at both the coronal and apical levels. However, the proportion of the distal movement was greater in the crown than in the root.
The stress distribution without attachments was greater mesial to the PDL. With the rectangular vertical attachment in which it was observed, abrupt changes were seen in the geometry of the attachment. With the beveled horizontal attachment, no strong stress concentration was observed. The optimized abutment showed smooth stress distribution in both the abutment and the tooth.
The greatest plastic deformation was found in the simulation without an attachment, decreased in the beveled horizontal and rectangular vertical attachments, and reached 0 mm in the optimized attachment.
Acknowledgment
We thank everyone who contributed to the development and accomplishment of the present investigation, your help was invaluable.
Ethical approval
The research/study complied with the Helsinki Declaration of 1964.
Declaration of patient consent
Patient’s consent not required as patient’s identity is not disclosed or compromised.
Conflicts of interest
The authors declare no conflicts of interest.
Use of artificial intelligence (AI)-assisted technology for manuscript preparation
The authors confirm that there was no use of artificial intelligence (AI)-assisted technology for assisting in the writing or editing of the manuscript and no images were manipulated using AI.
Financial support and sponsorship
Nil.
References
- A comparison of treatment effectiveness between clear aligner and fixed appliance therapies. BMC Oral Health. 2019;19:24.
- [CrossRef] [PubMed] [Google Scholar]
- Effect of composite attachment on initial force system generated during canine rotation with plastic aligners : A three-dimensional finite elements analysis. J Aligner Orthod. 2018;2:31-6.
- [Google Scholar]
- Effects of composite attachments on orthodontic clear aligners therapy: A systematic review. Materials(Basel). 2022;15:533.
- [CrossRef] [PubMed] [Google Scholar]
- Clinical effectiveness of Invisalign® orthodontic treatment: A systematic review. Prog Orthod. 2018;19:37.
- [CrossRef] [PubMed] [Google Scholar]
- The effectiveness of the invisalign appliance in extraction cases using the the ABO model grading system: A multicenter randomized controlled trial. Int J Clin Exp Med. 2015;8:8276-82.
- [Google Scholar]
- Four-premolar extraction treatment with invisalign. J Clin Orthod. 2006;40:493-500.
- [Google Scholar]
- Invisalign treatment of dental Class II malocclusions without auxiliaries. J Clin Orthod. 2010;44:665-72.
- [Google Scholar]
- Initial force systems during bodily tooth movement with plastic aligners and composite attachments: A three-dimensional finite element analysis. Angle Orthod. 2015;85:454-60.
- [CrossRef] [PubMed] [Google Scholar]
- A three-dimensional finite element analysis of upper-canine distalization with clear aligners, composite attachments, and class II elastics. J Clin Orthod. 2017;51:24-8.
- [Google Scholar]
- Scientific use of the finite element method in orthodontics. J Orthod. 2015;20:119-25.
- [CrossRef] [PubMed] [Google Scholar]
- Translation movements and torque control with smilers aligners: CBCT scan superimposition and root segmentation. J Aligner Orthod. 2018;2:307-16.
- [Google Scholar]
- Clear aligner orthodontic therapy of rotated mandibular round-shaped teeth: A finite element study. Angle Orthod. 2020;90:247-54.
- [CrossRef] [PubMed] [Google Scholar]
- Declaration of Helsinki, Ethical Principles for Scientific Requirements and Research Protocols Paris: World Medical Association; 2013. p. :29-32.
- [Google Scholar]
- Smart Force Features. 2015. Amsterdam: Align Technology BV; :5. Available from: https://www.aligntech.com/documents/Align%20Technology%20Corp%20Fact%20Sheet%202015%20Q4%20F.pdf [Last accessed on 2020 Sep 02]
- [Google Scholar]
- Effectiveness of an anterior mini-screw in achieving incisor intrusion and palatal root torque for anterior retraction with clear aligners. Angle Orthod. 2021;91:794-803.
- [CrossRef] [PubMed] [Google Scholar]
- Space closure using aligners. Dental Press J Orthod. 2020;25:85-100.
- [CrossRef] [PubMed] [Google Scholar]
- Forces and moments generated by aligner-type appliances for orthodontic tooth movement: A systematic review and meta-analysis. Orthod Craniofac Res. 2019;22:248-58.
- [CrossRef] [PubMed] [Google Scholar]
- Efficient design of a clear aligner attachment to induce bodily tooth movement in orthodontic treatment using finite element analysis. Materials (Basel). 2021;14:4926.
- [CrossRef] [PubMed] [Google Scholar]
- Optimal position of attachment for removable thermoplastic aligner on the lower canine using finite element analysis. Materials (Basel). 2020;13:3369.
- [CrossRef] [PubMed] [Google Scholar]
- How well does invisalign work? A prospective clinical study evaluating the efficacy of tooth movement with Invisalign. Am J Orthod Dentofac Orthop. 2009;135:27-35.
- [CrossRef] [PubMed] [Google Scholar]
- Effects of different aligner materials and attachments on orthodontic behavior. J Dent Sci. 2021;16:1001-9.
- [CrossRef] [PubMed] [Google Scholar]
- Three-dimensional finite element analysis on canine teeth distalization by different accessories of bracket-free invisible orthodontics technology. AIP Conf Proc. 2018;1955:30034.
- [CrossRef] [Google Scholar]
- Class II subdivision correction with clear aligners using intermaxilary elastics. Prog Orthod. 2018;19:32.
- [CrossRef] [PubMed] [Google Scholar]
- Staging orthodontic aligners for complex orthodontic tooth movement. Turk J Orthod. 2021;34:202-6.
- [CrossRef] [PubMed] [Google Scholar]
- Efficacy of clear aligners in controlling orthodontic tooth movement: A systematic review. Angle Orthod. 2015;85:881-9.
- [CrossRef] [PubMed] [Google Scholar]
- Effects of variable attachment shapes and aligner material on aligner retention. Angle Orthod. 2015;85:934-40.
- [CrossRef] [PubMed] [Google Scholar]
- In-vivo von mises strains during invisalign treatment. Am J Orthod Dentofac Orthop. 2010;138:399-409.
- [CrossRef] [PubMed] [Google Scholar]
- Torquing an upper central incisor with aligners-acting forces and biomechanical principles. Eur J Orthod. 2010;32:607-13.
- [CrossRef] [PubMed] [Google Scholar]
- Biomechanical effects of different auxiliary-aligner designs for the extrusion of an upper central incisor: A finite element analysis. J Healthc Eng. 2019;2019:9687127.
- [CrossRef] [PubMed] [Google Scholar]
- Three-dimensional modeling and finite element analysis in treatment planning for orthodontic tooth movement. Am J Orthod Dentofac Orthop. 2011;139:59-71.
- [CrossRef] [PubMed] [Google Scholar]