Translate this page into:
Immunohistochemical study on the postnatal growth changes of the spheno-occipital synchondrosis and tibial cartilage
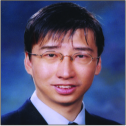
*Corresponding author: Kee-Joon Lee, Department of Orthodontics, Yonsei University College of Dentistry, 50-1 Yonsei-ro, Seodaemun-gu, Seoul 03722, Republic of Korea. orthojn@yuhs.ac
-
Received: ,
Accepted: ,
How to cite this article: Yoon T, Park S, Kwon M, Lee K. Immunohistochemical study on the postnatal growth changes of the spheno-occipital synchondrosis and tibial cartilage. APOS Trends Orthod 2019;9(3):149-55.
Abstract
Introduction:
The synchondrosis contributes to the growth of overall skull. The growth plate of the tibia is an analogous structure to the synchondrosis. The fibroblast growth factor receptors (FGFRs) are known to play an essential role in the proliferation and differentiation of cartilaginous cells.
Methods:
This study was purposed to examine the histological features of spheno-occipital synchondrosis (SOS) and tibial cartilage (TC) and the expression pattern of FGFR-1, -2 and proliferating cell nuclei antigen (PCNA) in SOS and TC of the postnatal mouse using an immunohistochemical method.
Results:
The width of SOS and TC reduced with age. The width of the SOS decreased, and then maintained, while the width of TC decreased gradually. Expression pattern of FGFRs indicated that they were involved in the postnatal bone growth and development. In SOS, FGFR-1 expression increased until the 14th day, and then, it showed a notable decrease. Comparing the level of expression, TC showed a stronger level than SOS at most stages. FGFR-2 showed in the resting and proliferating zones at an earlier stage of differentiation. With age, FGFR-2 expression reduced in previous zone and increased in the hypertrophic zone at both tissues. In PCNA study, cell proliferation was active in the resting and proliferating zone at an early stage. As mouse matured, the PCNA-positive cells usually expressed in the newly formed endosteal bone surface.
Conclusions:
This approach revealed a temporal and spatial change of FGFR-1, -2 expressions. The expression pattern of PCNA indicated that the chondrocytes of TC had more active metabolism than SOS at most of the stages. We can also speculate that FGFR-1 is a negative regulator of chondrogenesis, and FGFR-2 is a positive regulator of chondrogenesis.
Keywords
Fibroblast growth factor receptor
Cranial base
Synchondrosis
Tibia
Immunohistochemistry
INTRODUCTION
The bones develop and grow by two processes, endochondral and intramembranous ossification. The bones of the cranial base and tibia form by endochondral ossification. During endochondral ossification, a cartilage template forms first. Then, the cartilage template is vascularized, followed by recruitment of osteoclasts and osteoblasts, and then, the cartilage scaffold gradually replaced with bone. In the cranial base, the synchondrosis is the significant sites of growth and has a crucial role in determining the overall skull shape as well as relating each bone to its neighbor.[1-3] Furthermore, the size of the brain increased rapidly in the early age[4]. The cranial base protects and supports the brain, and the synchondroses play an important role in integrating the bones composing cranial base during the growth of brain.[5] Especially, the spheno- occipital synchondrosis (SOS) is important as it remains patent the longest between other synchondroses and provides significant growth in adolescence. In lower legs, the growth plate in the tibia is responsible for the longitudinal growth through an elaborately controlled process.[6]
Histologically, both of the synchondrosis and growth plate in tibia divide into three zones and, in each zone, the chondrocytes are at different maturation and metabolic stages.[7-9] At the resting zone, the cells (or chondrocytes or chondrogenic progenitor cells) are relatively in an inactive state; and then, they activate and differentiate to chondrocytes entering into the proliferative phase at the proliferating zone. Then, the chondrocytes begin to increase in size, and they begin to synthesize collagens and form the hypertrophic zone, where at the lower part calcification and mineralization of the extracellular matrix allows.[10] At their terminal differentiation stage, hypertrophic chondrocytes terminate the life cycle by apoptosis. The calcified zone of hypertrophic cartilage is invaded by blood vessels, bringing in the bone- forming cells (osteoblasts) and resorbing cells (osteoclasts), which will remodel and convert the mineralized cartilage into the trabecular bone.
Fibroblast growth factors (FGFs) consist of a family of at least 22 heparin-binding-related proteins and are major regulators of embryonic bone development.[11,12] FGFs bind to a family of FGF receptors (FGFRs) with high affinity. Four FGFRs (FGFR-1, -2, -3, and -4) are well known up to recently. The structure of the FGFRs includes (1) an extracellular domain, which consists of three immunoglobulins (Ig)- like domains, (2) a transmembrane domain, and (3) an intracellular tyrosine kinase domain, responsible for receptor dimerization and cell signaling.[13] The 3rd Ig loop or the linker region between 2nd and 3rd Ig loop in the Ig-like domain is the major mutation site in FGFRs. Kinase activates when the FGF ligand forms a trimolecular complex with FGFR and heparan sulfate proteoglycans.[14-18] Mutations in FGFR-encoding genes have been identified to cause craniosynostoses such as Apert, Pfeiffer, Jackson–Weiss, and Crouzon syndromes.[19,20] These FGFRs differ in their ligand specificity[21] and tissue distribution.[22,23] For example, in calvaria bones, FGFR-1 is mainly expressed in differentiated osteoblasts, while FGFR- 2 expressed in pre-osteoblasts. The principal function of FGFRs is in cellular behavior, such as cell proliferation and differentiation. The isoforms of the FGFR-1, -2, and -3 have been expressed at a specific area in the developing cranial bones, implicating its key role in the proliferation and differentiation for the cartilage growth.[24] Proliferating cell nuclear antigen (PCNA) clarifies the alternation process in cells and can be used as a marker for immunohistochemical analysis.
The purpose of this study was to investigate the postnatal developmental progression of the SOS and tibial cartilage (TC). For this purpose, the present study evaluated the histological changes on SOS and TC by age in postnatal mouse and investigated the expression of FGFR-1, -2, and PCNA which are used to identify the proliferating pattern of chondrocytes.
MATERIALS AND METHODS
Tissue preparation
Postnatal ICR mice at 1 day, 7 days, 14 days, and 21 days of age were used in this study. This study was approved by the Animal Care and Use Committee, Yonsei Medical Center. The cranial bases and tibias of the mouse were dissected and stored in ice-cold phosphate-buffered saline (PBS). Following overnight fixation at 4°C in 4% paraformaldehyde in PBS (pH 7.4), they were decalcified with 8% formic acid for 1 day to 10 days, depending on their age. The decalcified tissues were dehydrated and embedded in paraffin. Paraffin sections were cut at 6 µm thickness in the mid-sagittal plane, in parallel to the longitudinal axis of the cranial base and tibia by a microtome and mounted on silane-coating slide glasses [Figure 1]. The slide glasses were dried overnight at room temperature.

- Mid-sagittal section of cranial base and tibia. In preparation for immunohistochemical stain, the skin and associated tissues were removed from the cranial base (a) and tibia (b). The line and square stand for the axis of the section. Black arrow indicates the spheno-occipital synchondrosis.
Immunohistochemical staining
Immunohistochemical staining was performed on the dewaxed paraffin section. The midsagittal paraffin sections were deparaffinization and rehydration to facilitate the immunological reaction of antibodies with antigens in fixed tissue. Pretreatment was performed using a pressure cooker for up to 7 min in citrate buffer and then cooled in the room temperature for 15 min. Next, the slides were treated with 0.15 M ethylenediaminetetraacetic solution for 10 min. For enzyme digestion, the slides were placed in 0.2% hyaluronidase (Sigma, ST. Louis, MO, USA) solution at the 37°C container for 20 min. After washing procedures, the slides were transferred into 10% hydrogen peroxide/methanol for 10 min. Blocking solution (Zymed, San Francisco, CA, USA) was applied to the specimens for 15 min to reduce non-specific binding. After washing several times, the sections were incubated with rabbit polyclonal primary antibodies against FGFR-1, FGFR-2, and PCNA (Santa Cruz Biotechnology, Santa Cruz, CA, USA) diluted at 1:100, respectively, at 4°C cold room for overnight. After washing several times in PBS, the specimens were treated with a biotinylated anti-rabbit secondary antibody (Zymed, San Francisco, CA, USA) for 20 min at room temperature, and then, streptavidin HRP (Zymed, San Francisco, CA, USA) was conjugated on the secondary antibodies. The specimens were subsequently treated with diaminobenzidine tetrahydrochloride (Zymed, San Francisco, CA, USA) to visualize immunoreaction. And then, they were counterstained with Mayer’s Hematoxylin (Lab Vision, Fremont, CA, USA), dehydrated, and mounted with cover slide.
Histomorphometric analysis
All sections were photographed with a Leica DC 300F digital camera (Leica, Wetzlar, Germany). Image-Pro plus analyzer program (Media Cybernetics, Rockville, MD, USA) was used for measurement. The width of each zone was measured [Figure 2]. The measurement was done three times, and the mean values were used. The results of the immunohistochemical analysis were rated such as (+) weak intensity, (++) moderate intensity, and (+++) strong intensity.

- Distinct zones of spheno-occipital synchondrosis and tibia cartilage. Sphenooccipital synchondrosis (a) and tibia cartilage (b) can divide into three zones through cell shape. The cell of the resting zone is a round shape; the proliferating zone is a flattened shape; the hypertrophic zone is increased cell size. (photographed at ×400).
RESULTS
The histologic morphology of SOS and TC [Figure 3]
The width of SOS and TC was reduced over time. There was a reduction at all zones, resting, proliferating, and hypertrophic zone. The reduction of width in SOS had happened remarkably between 1st day and 7th day, especially at the hypertrophic zone and resting zone. And after 14th day, there was little change in the width. On the other hand, the width of TC was reduced gradually in every zone with age.

- Width changes at spheno-occipital synchondrosis and tibial cartilage. The width changes in spheno-occipital synchondrosis (SOS) and tibia cartilage (TC). There was a reduction in all zones. In SOS, the width was remarkably reduced at the hypertrophic zone between 7th day and 14th day. The width of TC was reduced smoothly compared to SOS. RZ = Resting zone, PZ = proliferating zone, HZ = Hypertrophic zone (length unit is μm).
Expression pattern of FGFR-1 in SOS and TC [Figure 4]

- Localization of fibroblast growth factor receptors (FGFR)-1 in spheno-occipital synchondrosis (SOS) and tibial cartilage (TC) at postnatal 1st day, 7th day, 14th day, and 21st day. Two images in the center are the sagittal view of cranial base (a) and tibia (b) stained with hematoxylin and eosin (HE) stain. The small rectangles in panel indicate the area enlarged SOS and TC. In the SOS (left column), FGFR-1 was localized in all zones, especially in the newly formed endosteal bone surfaces. The FGFR-1-positive cells were reduced in resting and proliferating zones; however, they were increased in the hypertrophic zone and newly formed bone surface with age. In the tibia (right column), localization of FGFR-1 was shown a similar pattern with SOS. However, the level of expression pattern in the TC was stronger than spheno-occipital synchondrosis at an early stage. (SOS: ×400, TC: ×200) +++: intensity of stain is strong, ++: moderate, +: weak.
FGFR-1 was expressed during development of the SOS and TC at all zones.
In SOS, FGFR-1 was localized mostly in the resting and proliferating zones at the 1st and 7th day. At 14th day, FGFR- 1 was localized broadly at all three zones with moderate-to- strong intensity. At 21 days, the FGFR-1 was expressed weak.
In TC, FGFR-1 was strongly localized in all three zones on the 1st day. From the 14th day, FGFR-1 was typically expressed in the hypertrophic zone and newly formed endosteal bone surface. At the 21st day, the localization pattern was similar to the 14th day pattern.
Expression pattern of FGFR-2 in SOS and TC [Figure 5]

- Localization of FGFR-2 in spheno-occipital synchondrosis (SOS) and tibial cartilage (TC) at postnatal 1st day, 7th day, 14th day, and 21st day. Two images in the center are the sagittal view of the cranial base (a) and tibia (b) stained with HE stain. The small rectangles in panel indicate the area enlarged SOS and TC. In SOS (left column), FGFR-2-positive cells were smoothly reduced in the proliferating zone with age. In TC (right column), localization of FGFR-2 was shown a similar pattern with SOS. However, the level of expression pattern in TC was stronger than SOS at the early stage. (SOS: ×400, TC: ×200) +++: intensity of stain is strong, ++: moderate, +: weak.
FGFR-2 was also presented during the development of SOS and TC in all zones.
In SOS, FGFR-2 was localized mostly in the resting and proliferating zones at the 1st and 7th day. From the 14th day, the FGFR-2 expression pattern was increased at proliferating and hypertrophic zones. At the 21st day, the intensity was the strongest.
In TC, FGFR-2 was observed in all three zones at the 1st and 7th day. From the 14th day, the expression pattern of FGFR-2 at resting and hypertrophic zones showed moderate-to-strong intensity. At the 21st day, FGFR-2 was strongly expressed in the proliferating zone.
Expression patterns of PCNA in SOS and TC [Figure 6]

- Localization of proliferating cell nuclei antigen (PCNA) in spheno-occipital synchondrosis (SOS) and tibial cartilage (TC) at postnatal 1st day, 7th day, 14th day, and 21st day. Two images in the center are the sagittal view of the cranial base (a) and tibia (b) stained with HE stain. The small rectangles in panel indicate the area enlarged SOS and TC. In SOS, the expression level was most active at 14 days and least active at 21 days. The PCNA-positive cells were increased in the bone surface with age. In TC, PCNA-positive cells were expressed at all three zones. The level of expression was reduced with age. As mouse matured, most of PCNA-positive cells were expressed in the bone surface. (SOS: ×400, TC: ×200) +++: intensity of stain is strong, ++: moderate, +: weak.
PCNA was presented during the development of the SOS and TC at all zones.
In SOS, PCNA was localized mostly in the resting and proliferating zones at the 1st and 7th day. At the 14th day, PCNA was observed remarkably in all zones, especially in the hypertrophic zone. At the 21st day, PCNA-positive cells were mostly found in the hypertrophic zone and the endosteal bone surface.
In the TC, PCNA was observed in the resting and proliferation zone at the 1st day. At the 7th day, PCNA was observed in proliferating and hypertrophic zone. From 14th day, the level of PCNA expressed in the hypertrophic zone and newly formed endosteal bone surface. At 21st day, PCNA was rarely observed.
DISCUSSION
The importance of the cranial base and tibia in the development
During the fetal period, ossification centers are formed in the cranial base synchondrosis and TC. In the postnatal period, endochondral ossification of the synchondrosis and TC contributes to the expansion of the ossification centers and growth of the cranial base and tibia. By analyzing the time course of normal growth and development of the endochondral cranial base comparing with a tibia of a mouse, the function of the cranial base and tibia growth in terms of endochondral ossification during the postnatal period was identified. There are few papers compared the development and growth of cranial base with TC during the postnatal period. In that respect, this experiment can be meaningful to identify the growth of the cranial base and TC during the postnatal period.
Involvement of the FGFR-1 and -2 in the endochondral ossification of the SOS and TC
In the endochondral bone formation, FGFR-1, -2 isoforms are all present during its development. It has been known that there are two major splicing isoforms in the FGFRs, namely b-form and c-form. Previous studies showed that Fgfr2c transcripts were detected in the resting and proliferating zone of chondrocytes as well as osteoblasts in the newly formed endosteal bone surface of the cranial base synchondrosis.[24,25] Our data also show a similar pattern to previous studies that FGFR-1, -2 may be involved in the growth of cranial base and TC growth during the postnatal period. From the comparison with preview studies, it can be speculated that the FGFRs observed in the present study are linked to being the c-isoform.
In immunohistochemical staining, localization of FGFR-1 was mainly seen in the resting and proliferating zones at an early stage in SOS and all zones in TC. At a later stage, the FGFR-1 was upregulated at a hypertrophy zone.
In SOS, there seems to be a connection between the FGFR-1 and the width of zones. After 14th day, the intensity of FGFR- 1 remarkably reduced, and the width was almost maintained. On the other hand, the width had decreased gradually in TC, where the FGFR-1 expression had increased. It can be assumed that brain growth may cause this difference. However, further study is needed to investigate the causative factors.
Localization of FGFR-2 in SOS and TC was seen in the resting and proliferating zone at an early stage. This result overlaps with mRNA localization of FGFR-1 and FGFR - 2 using fetal mice and localization FGFR-1 and FGFR-2 at the postnatal growth plate.[26,27] These results indicate the possibility that the FGFRs may be involved in the particular stages of chondrocyte proliferation and differentiation during growth. Deletion of Fgfr2c or expression of gain-of-function mutations in Fgfr2c in mice results in craniofacial and tibia abnormalities.[28,29] It was also shown that a dominant mutation that affects alternative splicing in FGFR-2 caused synostosis in mice.[30] Synchondrosis fusion and developmental deficiency in the basicranium of Fgfr2c null mice imply primary anomalies in the basicranium, simultaneously in the cranial vault. Loss-of-function mutations in FGFRs are not well known. Embryo lacking FGFR-1 or -2 dies before skeletal development.[31,32] In another study, expression of FGFR-1 in the hypertrophic chondrocyte suggests a role in the survival of the hypertrophic chondrocyte, in regulating a feedback signal to control the rate of differentiation, in regulating the production of the unique extracellular matrix products of these cells or in signaling their eventual apoptotic death.[33] In our study, the FGFR-1 and FGFR-2 were predominantly expressed both in the cartilage phase and in the new bone phase. These findings imply that the FGFR-1 and FGFR-2 may be involved in endochondral ossification and osteogenesis in the chondrogenesis phase, especially in the hypertrophy zone by activation of cell proliferation and differentiation. We can suggest that FGFR-1 expressed in the hypertrophy zone is thought to a negative regulator of chondrogenesis and controls the differentiation of the hypertrophy cells in the cartilage. Furthermore, we can expect that FGFR-2 expressed in the resting and proliferating zone is a positive regulator of chondrogenesis and control the proliferation and differentiation of chondrocytes in the process of chondrogenesis.
Localization of the PCNA in SOS and TC during the development
In the endochondral bone formation, PCNA is all present during its development. Previous studies showed that PCNA- positive chondrocytes were found in all growth plates and all three zones, mostly in the lower proliferating and upper hypertrophic zones.[34] Our results were similar to previous findings. The PCNA-positive chondrocytes level was downregulated with age. In TC, the intensity was smoothly reduced with age. However, in the newly formed endosteal bone surface, the PCNA expression level was increased. Cell proliferation of TC was more active than SOS at the early stage, but at the later stage, the pattern was the opposite.
CONCLUSIONS
The purpose of this study was to investigate the postnatal developmental progression of the cranial base and tibia. Concerning the expression pattern of FGFR-1, -2 in the cranial base and tibia development, this study had shown that FGFR-1 and FGFR-2 were predominantly expressed both in the cartilage phase and in the bone phase. These findings imply that FGFR-1 and FGFR-2 may be involved in endochondral ossification and osteogenesis as well as chondrogenesis. Moreover, we can assume FGFR- 1 and FGFR-2 can control the cartilage through the cell proliferation of differentiation. FGFR-1 expressed in the hypertrophy zone is thought to be a negative regulator of chondrogenesis and control the differentiation of the hypertrophy cells in the cartilage. FGFR-2 expressed in the resting and proliferating zone is considered to be the positive regulators of chondrogenesis and control the proliferation and differentiation of chondrocytes in the process of chondrogenesis. In general, TC was sustained for a long time compared to SOS. With observing the localization of PCNA, we can suggest that the process of proliferation and differentiation in the TC is more active than SOS during early development.
Acknowledgments
This research was supported by Basic Science Research Program through the National Research Foundation of Korea funded by the Ministry of Education. (NRF-2017R1D1A1B03035435).
Declaration of patient consent
Patient's consent not required as patients identity is not disclosed or compromised.
Financial support and sponsorship
Nil.
Conflicts of interest
There are no conflicts of interest.
References
- The human SOS. I. The time of closure appraised macroscopically. Acta Odontol Scand. 1972;30:349-56.
- [CrossRef] [PubMed] [Google Scholar]
- Growth of the cartilages of the mid-line cranial base: A radiographic and histological study. J Anat. 1983;136:307-20.
- [Google Scholar]
- The growth of the cranial base in the wistar albino rat studied by vital staining with alizarin red S. Acta Odontol Scand Suppl. 1971;29:1-44.
- [Google Scholar]
- The basics of brain development. Neuropsychol Rev. 2010;20:327-48.
- [CrossRef] [PubMed] [Google Scholar]
- Relationships between cranial base synchondroses and craniofacial development: A review. Open Anat J. 2010;2:67-75.
- [CrossRef] [Google Scholar]
- Transcription factors in bone: Developmental and pathological aspects. Trends Mol Med. 2002;8:340-5.
- [CrossRef] [Google Scholar]
- Recent studies on the biological action of parathyroid hormone (PTH)-related peptide (PTHrP) and PTH/PTHrP receptor in cartilage and bone. Histol Histopathol. 2000;15:957-70.
- [Google Scholar]
- Parathyroid hormone-related peptide-depleted mice show abnormal epiphyseal cartilage development and altered endochondral bone formation. J Cell Biol. 1994;126:1611-23.
- [CrossRef] [PubMed] [Google Scholar]
- Programmed cell death of chondrocytes and aberrant chondrogenesis in mice homozygous for parathyroid hormone-related peptide gene deletion. Endocrinology. 1996;137:5055-67.
- [CrossRef] [PubMed] [Google Scholar]
- Systemic and local regulation of the growth plate. Endocr Rev. 2003;24:782-801.
- [CrossRef] [PubMed] [Google Scholar]
- Fibroblast growth factors as multifunctional signaling factors. Int Rev Cytol. 1999;185:45-106.
- [CrossRef] [Google Scholar]
- FGF signaling pathways in endochondral and intramembranous bone development and human genetic disease. Genes Dev. 2002;16:1446-65.
- [CrossRef] [PubMed] [Google Scholar]
- Structural and functional diversity in the FGF receptor multigene family. Adv Cancer Res. 1993;60:1-41.
- [CrossRef] [Google Scholar]
- Cloning and expression of two distinct high-affinity receptors cross-reacting with acidic and basic fibroblast growth factors. EMBO J. 1990;9:2685-92.
- [CrossRef] [PubMed] [Google Scholar]
- Complexity of FGF receptors: Genetic basis for structural diversity and functional specificity. FASEB J. 1992;6:3362-9.
- [CrossRef] [PubMed] [Google Scholar]
- Heparin-induced oligomerization of FGF molecules is responsible for FGF receptor dimerization, activation, and cell proliferation. Cell. 1994;79:1015-24.
- [CrossRef] [Google Scholar]
- Determination of ligand-binding specificity by alternative splicing: Two distinct growth factor receptors encoded by a single gene. Proc Natl Acad Sci U S A. 1992;89:246-50.
- [CrossRef] [PubMed] [Google Scholar]
- Fibroblast-growth-factor receptor mutations in human skeletal disorders. Trends Genet. 1995;11:308-13.
- [CrossRef] [Google Scholar]
- Skeletal disorders associated with fibroblast growth factor receptor mutations. Curr Opin Genet Dev. 1997;7:378-85.
- [CrossRef] [Google Scholar]
- Receptor specificity of the fibroblast growth factor family. J Biol Chem. 1996;271:15292-7.
- [CrossRef] [PubMed] [Google Scholar]
- Different members of the fibroblast growth factor receptor family are specific to distinct cell types in the developing chicken embryo. Dev Biol. 1993;155:107-23.
- [CrossRef] [PubMed] [Google Scholar]
- Expression of fibroblast growth factor receptors (FGFR1, FGFR2, FGFR3) in the developing head and face. Dev Dyn. 1997;210:41-52.
- [CrossRef] [Google Scholar]
- Fgfr mRNA isoforms in craniofacial bone development. Bone. 2003;33:14-27.
- [CrossRef] [Google Scholar]
- Negative autoregulation of fibroblast growth factor receptor 2 expression characterizing cranial development in cases of apert (P253R mutation) and pfeiffer (C278F mutation) syndromes and suggesting a basis for differences in their cranial phenotypes. J Neurosurg. 2001;95:660-73.
- [CrossRef] [PubMed] [Google Scholar]
- Fibroblast growth factor expression in the postnatal growth plate. Bone. 2007;40:577-86.
- [CrossRef] [PubMed] [Google Scholar]
- Two FGF receptor genes are differentially expressed in epithelial and mesenchymal tissues during limb formation and organogenesis in the mouse. Development. 1992;114:233-43.
- [Google Scholar]
- The IIIc alternative of fgfr2 is a positive regulator of bone formation. Development. 2002;129:3783-93.
- [Google Scholar]
- Conditional inactivation of FGF receptor 2 reveals an essential role for FGF signaling in the regulation of osteoblast function and bone growth. Development. 2003;130:3063-74.
- [CrossRef] [PubMed] [Google Scholar]
- A splicing switch and gain-of-function mutation in fgfR2-IIIc hemizygotes causes apert/Pfeiffer-syndrome-like phenotypes. Proc Natl Acad Sci U S A. 2001;98:3855-60.
- [CrossRef] [PubMed] [Google Scholar]
- Murine FGFR-1 is required for early postimplantation growth and axial organization. Genes Dev. 1994;8:3045-57.
- [CrossRef] [PubMed] [Google Scholar]
- Fgfr2 is required for limb outgrowth and lung-branching morphogenesis. Proc Natl Acad Sci U S A. 1999;96:11895-9.
- [CrossRef] [PubMed] [Google Scholar]
- Spatio-temporal expression of FGFR 1, 2 and 3 genes during human embryo-fetal ossification. Mech Dev. 1998;77:19-30.
- [CrossRef] [Google Scholar]
- Apoptosis and proliferation of growth plate chondrocytes in rabbits. J Bone Joint Surg Br. 1997;79:483-6.
- [CrossRef] [PubMed] [Google Scholar]